Air quality appraisal: impact pathways approach
Updated 2 March 2023
This guidance details the process for estimating the impact on air quality resulting from policies or projects. This method is called the impact pathways approach (IPA). Use it when the:
- air quality impacts are more than £50million
- main objective of the policy or project is changes in air quality
IPA is also used to produce air quality damage and activity costs - a set of pre-calculated values, expressed in cost per tonne (£/tonne) of emissions and cost per kilowatt hour (p/KWh) for activity costs. These values can be used instead of the IPA where air quality impacts are less than £50 million.
The damage cost guidance explains how to use damage costs. This impact pathways approach details the methodology underlying them.
The IPA updates are the result of a Department for Environment, Food and Rural Affairs (Defra) review to reflect improvements in air pollution modelling and the underpinning evidence base. This includes the addition of wider health impacts. The review focused on areas where developments in the evidence base had an impact on cost estimates.
1. Impact pathways approach stages
The IPA has 5 stages:
- There is a policy intervention.
- Model the dispersion of air pollutant emissions.
- From stage 2, understand changes in ambient pollutant concentrations in different locations (using dispersion modelling).
- Estimate how those changes in concentrations affect different impact pathways related to health, economy and environment (using concentration response functions).
- Value those impacts using a single monetary metric (using valuation techniques).
Using the IPA, Defra has calculated damage costs for the UK as a whole. The results are summarised by calculating the average value associated with one tonne of emissions for different pollutants and sectors.
This means when using the damage costs only to value air quality, skip steps 3 and 4 of the IPA approach. Find the change in emissions from the policy intervention, which is valued using the damage costs.
2. Dispersion
Start by estimating how changes in air pollution emissions (resulting from a policy or project) translate into changes in concentrations.
A range of dispersion models are available and specialist consultancies can provide this service. Email Defra [email protected] for advice on the most appropriate choice of model.
Defra estimates ambient concentrations of air pollutants for the UK as a whole using the Pollution Climate Mapping (PCM) model. The PCM model is an atmospheric dispersion model that translates emissions from various sources into concentration estimates on a 1km by 1km grid across the UK using:
- emissions data from the National Atmospheric Emissions Inventory (NAEI)
- meteorological data
- modelling of chemical processes
Current damage costs are based on emissions estimates from 2019. By combining the concentration estimates produced by the PCM with population data from the 2011 census (updated to be applicable for 2019), you get a population-weighted mean concentration for each pollutant. For further information on the PCM model, including details of the emissions sources that are included, see Brookes and others (2015).
The average relationship between emissions and exposure to concentrations is calculated as a population-weighted mean for a pollutant divided by the total annual emissions of that pollutant. This provides the basis for ‘national’ damage cost estimates.
Values are also provided for different sector and location types, since emissions from particular sources will differ in the number of people that are exposed to the resulting concentrations. For example, emissions in city centres will have a greater effect on exposure than emissions in rural areas. The damage costs are calculated based on the geographical locations of emissions sources in different sectors, as recorded in the NAEI.
The PCM model estimates concentrations for 5 key pollutants:
- particulate matter (PM)
- nitrogen dioxide (NOx)
- volatile organic compounds (VOCs)
- ammonia (NH3)
- sulphur dioxide (SO2)
There are also chemical interactions associated with some of these pollutants that you should account for in your dispersion modelling. In particular:
- emissions of NOx, NH3 and SO2 contribute to the formation of PM and NOx
- VOCs affect the formation of ozone (O3)
This means there are some pathways associated with O3 included in the damage costs even though there are no damage cost values for emissions of O3 on its own.
For the 2023 update to the damage cost values, dispersion modelling has been updated in a number of ways, including:
- the application of scaling factors to adjust non-road transport emissions from 2019 values to values suitable for use in dispersion modelling for 2020 to take account of the reduction in activity due to the coronavirus (COVID-19) pandemic
- the use of specific 2020 traffic counts
- updated assumption on vehicle speeds and a revised method for assignment of average speed based on speed limits
- use of meteorological data from the weather and research forecasting model for 50 km squares across the UK (previous assessments used observed meteorological data for a single location)
- routine updates and improvements to methods used to map the emissions estimates across the UK
Many of the pathways described in this method refer to PM2.5 emissions. Convert PM10 emissions into estimated PM2.5 emissions based on ratios between these 2 pollutants from the NAEI. Conversion factors for PM emissions from different sectors are in the damage cost guidance.
3. Impact pathways
Next, assess the changes in outcomes that result from population-weighted concentration changes estimated through dispersion modelling. These outcomes include impacts on:
- public health
- the natural environment
- the economy
Concentration response functions (CRFs) allow concentration changes to be translated into impacts. These functions express changes in outcomes per unit in concentrations (measured in micrograms per cubic metre, µg/m3). For example, a 1µg/m3 increase in population-weighted mean concentration of particulate matter leads to a 5% increase in the incidence of a particular disease.
Tables 4, 5 and 6 each of the concentration response functions that are used to estimate damage costs and recommended for use in any bespoke IPA.
The full list of health impact pathways is:
- mortality effects of long-term (or chronic) exposure
- mortality effects of short-term (or acute) exposure
- morbidity effects of short-term exposure: respiratory hospital admissions
- morbidity effects associated with long-term (chronic) exposure. These are ischaemic heart disease, stroke, lung cancer, asthma (in adults and children) and chronic bronchitis, diabetes
The full list of environment impact pathways is:
- damage caused by sulphur dioxide to materials
- damage caused by ozone to materials (rubber)
- soiling of buildings due to PM
- ecosystem damages
Economic impacts on productivity through human health effects are also included in this methodology.
3.1 Health impacts
The health impact pathways and CRFs that are included in this IPA are selected based on a combination of advice from:
These are impacts where there’s at least reasonable evidence of an association with exposure to air pollutant concentrations. For some impacts there’s only weak or emerging evidence of an association – where possible, these impacts are included only in the high estimates of damage costs for use in sensitivity analyses.
Tables 1 to 3 show the full range of primary health impact pathways that are included for each pollutant.
3.2 Table 1: PM impact pathways
Impact pathway (PM) | Low | Central | High |
---|---|---|---|
Chronic mortality | Yes | Yes | Yes |
Respiratory hospital admissions | Yes | Yes | Yes |
Cardiovascular hospital admissions | No | No | Yes |
Ischaemic heart disease | Yes | Yes | Yes |
Stroke | Yes | Yes | Yes |
Lung cancer | Yes | Yes | Yes |
Asthma (children) | Yes | Yes | Yes |
Chronic bronchitis | No | No | Yes |
Diabetes | No | No | Yes |
3.3 Table 2: NOx impact pathways
Impact pathway (NOx) | Low | Central | High |
---|---|---|---|
Chronic mortality | Yes | Yes | Yes |
Asthma (children) | Yes | Yes | Yes |
Respiratory hospital admissions | Yes | Yes | Yes |
Asthma (adults) | No | No | Yes |
Diabetes | No | No | Yes |
Lung cancer | No | No | Yes |
3.4 Table 3: SO2 impact pathways
Impact pathway (SO2) | Low | Central | High |
---|---|---|---|
Acute mortality | Yes | Yes | Yes |
Respiratory hospital admissions | Yes | Yes | Yes |
There are no health impact pathways included which associate a health impact directly with exposure to ammonia or VOCs, however effects are captured through their secondary effect on the formation of other air pollutants in the atmosphere. For example, VOCs and NOx have effects on the formation of ozone, which is associated with acute mortality and hospital admissions.
3.5 Mortality pathways
The chronic mortality pathway is a measure of all-cause mortality – this means the pathway is based on an estimate of the relationship between chronic exposure to air pollutants and premature deaths, regardless of the conditions (for example respiratory, cardiovascular) that may have preceded death.
The impact of a one-year change in emissions on all-cause mortality associated with long-term exposure is calculated using life tables (which you will need to undertake if conducting your own IPA analysis). This technique simulates changes in survival rates at different ages for the UK population and estimates total life years lost due to a one-year change in emissions. This approach assumes that a sustained change in emissions (greater than one year) can be estimated by the sum of consecutive one-year changes in emissions.
Acute mortality refers to loss of life due to short-term impacts of air pollution. For example, a person suffering with an existing respiratory condition may be more likely to experience a fatal episode in periods of particularly poor air quality. This is in contrast to the long-term effects of air pollution on human health that are captured in the chronic mortality pathway.
3.6 Morbidity pathways
The ‘morbidity pathways’ (all pathways except mortality associated with chronic or acute exposure) account for air pollution causing a reduction in the quality of life as well as total years lived. Concentration response functions for these pathways are recommended by COMEAP and PHE. For most of the morbidity pathways (ischaemic heart disease, stroke, diabetes, lung cancer and asthma) concentration response functions estimate the change in incidence of conditions related to air pollution. That’s the changes to the flow of new cases (‘incidence’) rather than changes to the stock of existing cases (‘prevalence’).
To estimate damage associated with a one-year change in emissions, calculate the costs of an incident case over the lifetime of an individual. That means valuing each year of the incident case over the average number of years that a person lives with the disease.
The chronic bronchitis pathway is estimated using a prevalence approach, following COMEAP recommendations. Concentration response functions for hospital admissions are also based on COMEAP advice.
Cardiovascular hospital admissions should not be estimated under a low and central sensitivity scenarios due to the potential overlap with chronic cardiovascular conditions. The current approach to quantifying cardiovascular hospital admissions attributable to short-term exposures to PM2.5 are based on coefficients from time-series studies. It is not clear to what extent these avoided hospital admissions are a subset of the cardiovascular benefits of reductions in long-term exposure to PM2.5. The central estimate for cardiovascular hospital admissions is now only included in the high damage cost sensitivity scenario.
There is also the potential for overlap between chronic respiratory conditions and respiratory hospital admissions. However, the risk of overlap is considered lower for respiratory relative to cardiovascular conditions, in particular given respiratory conditions associated with chronic exposure, such as chronic bronchitis and asthma in adults, are only included in the high sensitivity damage cost. In addition, hospital admissions are still a small effect. As such respiratory hospital admissions can be assessed in central (and low) scenario analysis.
3.7 Environmental impacts
Air pollutants can have a range of negative impacts on the environment and ecosystems. For your policy or project there may only be specific pathways that are relevant. Conduct a bespoke analysis of potential environmental and ecosystem impacts resulting from the decision being appraised.
Four environmental impact pathways are included in the impact pathways methodology used to work out damage costs:
- damage caused by sulphur dioxide to buildings
- damage caused by ozone to materials
- soiling of buildings due to PM
- ecosystem damages
Emissions of sulphur dioxide are the main cause of acid rain. The analysis of these impacts was advanced through work by the Europe-wide International Co-operative Programme Materials (2003) and quantification under various studies for the European Commission DG Research, in particular ExternE and associated projects.
Ozone can have a damaging effect on materials such as rubbers and paints. This impact is included in damage costs using evidence from a study by Holland and others (1998). This pathway is a secondary mechanism included in damage costs for NOx and VOCs due to their impact on the formation of ozone in the atmosphere.
Particulate matter can cause blackening on the exterior of buildings, which creates costs from reduced amenity and increased cleaning. This impact is included in damage costs using evidence from a study by Rabl and others (1998).
A range of impacts on ecosystems has been quantified in a Defra-commissioned study by Jones and others (2014). The study includes impacts on agricultural production and recreational disamenity. This study provides national average costs associated with emissions of different pollutants. In the absence of local information on ecosystem impacts, you can incorporate these values (expressed in £ per tonne of emissions) into an impact pathway approach analysis.
3.8 Economic impacts
Air pollution affects the economy by reducing the ability of workers to attend the workplace and to be productive in their work.
This impact is included in the damage costs based on a method developed by Ricardo AEA (2014). The effect on productivity is estimated through a range of pathways including, for example, mortality. However only 2 pathways from this research are included in this method to avoid overlap:
- absenteeism and work-days lost for employees, volunteers and carers (PM2.5)
- presenteeism and minor restricted activity days for employees (PM2.5 and O3)
These impacts are included in the central and high scenarios in the damage costs.
3.9 Concentration response functions (CRF)
Concentration response functions (CRFs) are used to estimate the impacts of air pollution. CRFs link a change in exposure to a pollutant to its consequent impacts by expressing a change in a health or non-health outcome for a given change in pollutant concentrations. The CRFs used in this approach, which have been outlined in the below table, were taken from an extensive underlying literature on health effects of air pollution and follow the latest recommendations of COMEAP.
The low, central and high in the following tables does not refer to the low, central or high sensitivity scenarios associated with the IPA approach. It refers to the uncertainty range surrounding the CRFs themselves.
3.10 Table 4: CRF relative risk (% change per 10µg/m3 change in pollutant)
Pollutant | Pathway | Low | Central | High |
---|---|---|---|---|
PM2.5 | Mortality (long-term exposure) | 6 | 8 | 9 |
PM2.5 | Respiratory hospital admission | -0.63 | 0.96 | 2.58 |
PM2.5 | Cardiovascular hospital admission | 0.26 | 0.9 | 1.53 |
SO2 | Mortality (short-term exposure) | 0.6 | 0.6 | 0.6 |
SO2 | Respiratory hospital admission | 0.5 | 0.5 | 0.5 |
O3 | Mortality (short-term exposure) | 0.12 | 0.34 | 0.56 |
O3 | Respiratory hospital admission | 0.3 | 0.75 | 1.2 |
O3 | Cardiovascular hospital admission | -0.06 | 0.11 | 0.27 |
NO2 | Respiratory hospital admission | 0.33 | 0.57 | 0.82 |
NO2 | Mortality (long-term exposure)* | 0.8 | 2.3 | 3.7 |
PM2.5 | Diabetes | 2 | 10 | 18 |
PM2.5 | Lung cancer | 4 | 9 | 14 |
NO2 | Diabetes* | 2 | 5 | 7 |
NO2 | Lung cancer* | 0 | 2 | 3 |
*These CRFs have been reduced to 40% of their full value, to account for confounding effects of other pollutants
3.11 Table 5: CRF hazard ratio (% change per 10µg/m3 change in pollutant)
Pollutant | Pathway | Low | Central | High |
---|---|---|---|---|
PM2.5 | IHD | -1 | 7 | 16 |
PM2.5 | Stroke | -1 | 11 | 25 |
*These CRFs have been reduced to 40% of their full value, to account for confounding effects of other pollutants
3.12 Table 6: CRF odds ratio (change in baseline odds for 10µg/m3 change in pollutant)
Pollutant | Pathway | Low | Central | High |
---|---|---|---|---|
PM10 | Chronic bronchitis | 1.02 | 1.32 | 1.71 |
PM2.5 | Asthma (older children) | 1.22 | 1.48 | 1.97 |
NO2 | Asthma (adults)* | 0.996 | 1.04 | 1.08 |
NO2 | Asthma (small children)* | 1.01 | 1.08 | 1.12 |
NO2 | Asthma (older children)* | 1 | 1.03 | 1.06 |
*These CRFs have been reduced to 40% of their full value, to account for confounding effects of other pollutants
3.13 Relative risk, hazard ratio and odds ratio
The estimation of the incidence change due to a decrease of 1 μg/m3 of PM2.5 or NO2 is different depending on whether the CRF is based on the relative risk, hazard ratio or odds ratio. The change in incidence (ΔIi) per 100,000 inhabitants when the CRF is based on either the relative risk or hazard ratio, is estimated as the:
- product between the change in concentration of the pollutant
- baseline incidence
- population
- as in Equation 1:
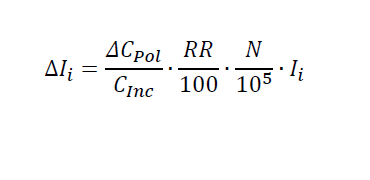
Equation 1: the change in incidence (ΔIi) per 100,000 inhabitants when the concentration response function is based on either the relative risk or hazard ratio
The change in incidence of disease i (the disease in question), is equal to:
- The change in concentration of a given pollutant, divided by the concentration increment on which the CRF is based (5 or 10 μg/m3).
- Multiplied by the relative risk (or hazard ratio, if applicable), divided by 100.
- Multiplied by the total population of the UK, divided by 10 to the power of 5.
- Multiplied by the age and gender-weighted incidence of disease i.
Where:
- ΔCPol is the change in concentration of a given pollutant (PM2.5, NO2)
- CInc is the concentration increment on which the CRF is based (5 or 10 μg/m3)
- RR is the Relative Risk (or Hazard Risk, if applicable)
- N is the total population of the UK
- Ii is the age and gender-weighted incidence of a disease i
The estimation of the change in incidence (ΔIi) per 100,000 inhabitants is more complex when the CRF is based on the odds ratio (OR). You must estimate the odds of reporting the disease at the new concentration (κi) first, as in Equation 2:
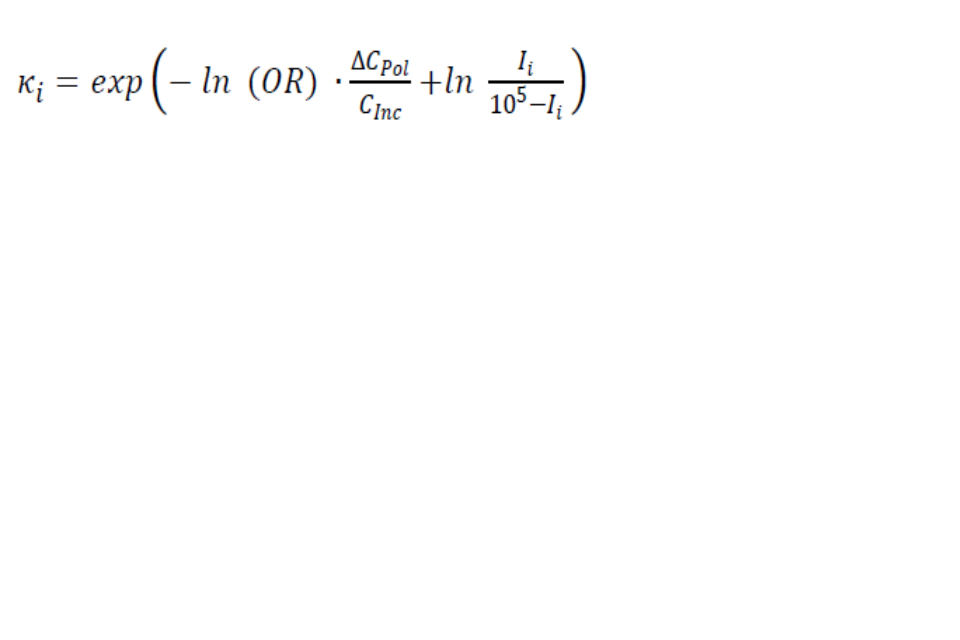
Equation 2: the odds of reporting the disease i at the new concentration response function
Odds of reporting the disease i at the new concentration is equal to the exponential function of: 1. The negative natural logarithm of the odds ratio. 2. Multiplied by the change in concentration of a given pollutant, divided by the concentration increment on which the CRF is based (5 or 10 μg/m3). 3. Plus the natural logarithm of the fraction of: age and gender-weighted incidence of disease i, divided by 10 to the power 5 minus the age and gender-weighted incidence of disease i.
Then estimate the change in incidence (ΔIi) per 100,000 inhabitants as a function of the odds of reporting the disease at the new concentration (κi) as in Equation 3:
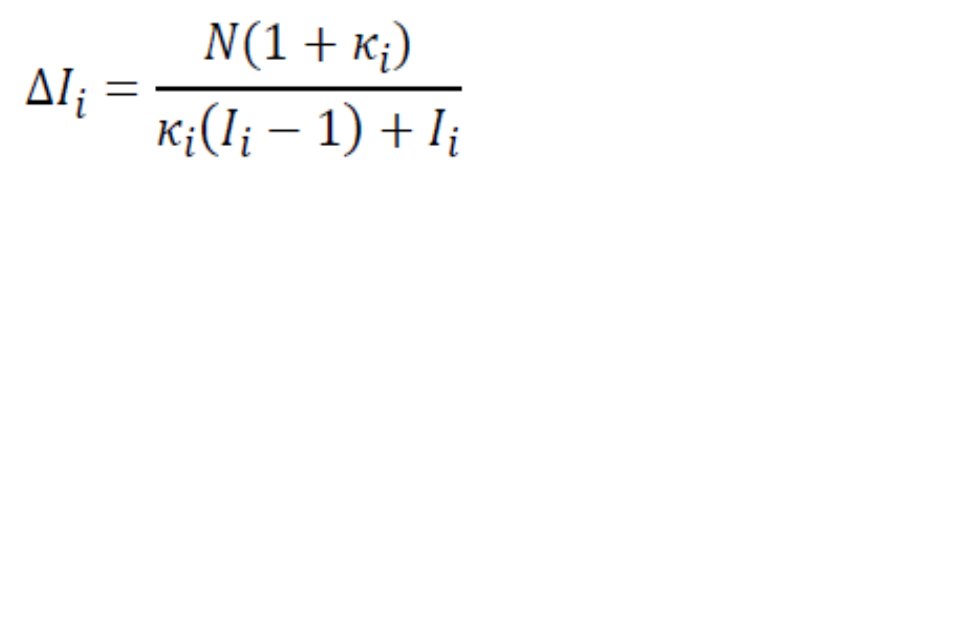
Equation 3: the change in incidence of disease
The change in incidence of disease is equal to the fraction:
Numerator
- The total population of the UK.
- Multiplied by one plus the odds of reporting the disease i at the new concentration (found in equation 2).
Denominator
- The odds of reporting the disease i at the new concentration (found in equation 2).
- Multiplied by the age and gender-weighted incidence of a disease i, minus one.
- Plus the age and gender-weighted incidence of a disease i.
3.14 Table 7: pathways included for each pollutant
This table shows which pathways are included for each pollutant and the type of impact, where:
- P denotes a primary effect - that’s a direct impact (for example SO2 emissions have a direct impact on material damage)
- 2 denotes a secondary effect - that is an indirect impacts (for example SO2 emissions have a secondary impact on cardiovascular hospital admissions)
- *denotes where a pathway is only included within the ‘high’ scenario and not in the central or low scenarios
Pathway | Pollutant | Damage cost of PM2.5 | Damage cost of NOx | Damage cost of SO2 | Damage cost of NH3 | Damage cost of VOC |
---|---|---|---|---|---|---|
Mortality (long-term exposure) | PM2.5 | P | 2 | 2 | 2 | n/a |
Mortality (long-term exposure) | NO2 | n/a | P | n/a | n/a | n/a |
Respiratory hospital admission | PM2.5 | P | 2 | 2 | 2 | n/a |
Respiratory hospital admission | NO2 | n/a | P | n/a | n/a | n/a |
Respiratory hospital admission | SO2 | n/a | n/a | P | n/a | n/a |
Respiratory hospital admission | O3 | n/a | 2 | n/a | n/a | 2 |
Chronic bronchitis | PM10 | P* | 2* | 2* | 2* | n/a |
IHD | PM2.5 | P | 2 | 2 | 2 | n/a |
Asthma (Adults) | NO2 | n/a | P* | n/a | n/a | n/a |
Asthma (Children) | PM2.5 | P | 2 | 2 | 2 | n/a |
Asthma (Small children) | NO2 | n/a | P | n/a | n/a | n/a |
Asthma (Older children) | NO2 | n/a | P | n/a | n/a | n/a |
Stroke | PM2.5 | P | 2 | 2 | 2 | n/a |
Diabetes | PM2.5 | P* | 2* | 2* | 2* | n/a |
Diabetes | NO2 | n/a | P* | n/a | n/a | n/a |
Lung cancer | PM2.5 | P | 2 | 2 | 2 | n/a |
Lung cancer | NO2 | n/a | P* | n/a | n/a | n/a |
Deaths brought forward (mortality – short-term exposure) | SO2 | n/a | n/a | P | n/a | n/a |
Deaths brought forward (mortality – short-term exposure) | O3 | n/a | 2 | n/a | n/a | 2 |
Material damage | SO2 | n/a | n/a | P | n/a | n/a |
Material damage | O3 | n/a | 2 | n/a | n/a | 2 |
Building soiling | PM10 | P | n/a | n/a | n/a | n/a |
Productivity | PM2.5 | P | 2 | 2 | 2 | n/a |
Productivity | NO2 | n/a | P* | n/a | n/a | n/a |
Productivity | O3 | n/a | 2* | n/a | n/a | 2* |
Ecosystems | NO2 | n/a | P | n/a | n/a | n/a |
Ecosystems | O3 | n/a | 2 | n/a | n/a | 2 |
Ecosystems | NH3 | n/a | n/a | n/a | P | n/a |
4. Valuation
Having identified and quantified the physical impacts of unit changes in emissions of different pollutants, each of these impacts is monetised using a range of different methods.
4.1 Mortality associated with long-term exposure
The value of life years lost due to the chronic effects of air pollution are monetised using values estimated in a study by Chilton and others (2004). This research involved a bespoke contingent valuation survey of over 600 people in England, Scotland and Wales. Respondents were asked to indicate their willingness to pay for reductions in the health effects associated with air pollution, including reduced life expectancy.
The value of a life year lost due to the effect of air pollution obtained from this study has been rebased to 2022 prices and is used to monetise the all-cause mortality pathway in damage cost calculations. The value is £50,600 (2022 prices) and is based on life years lost being in normal health.
4.2 Mortality associated with short-term exposure
Life years lost due to the acute effects of short-term exposure to air pollution are valued at £26,200 per life year lost. Each death brought forward due to acute exposure is assumed to incur 2 to 6 months of lost life (4 months in the central scenario) in poor health.
4.3 Morbidity
The standard method for monetising the loss of quality of life due to health conditions is ‘Quality Adjusted Life Years’ (QALYs). This is different from the value of a life year used in the mortality pathway since the method for valuing morbidity involves assigning a utility weight to different conditions: a QALY value of 1 is attached to a life year lived in perfect health since and values that approach 0 indicate increasingly poor quality of life.
In accordance with the Green Book, and is valued at £72,000 in 2022 prices.
The utility weights (QALY values) used in calculating damage costs are from Sullivan and others (2011). The product of this utility weight and the perfect health value (£72,000 in 2022 prices) is the value given to the loss of quality of life for one year due to a particular condition. The total value of each morbidity pathway is the annual cost of lost quality of life combined with the average duration of disease.
The average duration of disease measures the mean number of years that a person will experience a disease following initial incidence. Duration of the disease ends through:
- remission
- death from the disease
- death from all other causes
To calculate damage costs, work out the average duration values using:
- the DISMOD II model
- epidemiological input data from PHE’s modelling of costs to the NHS resulting from air pollution
Table 6 details the average duration of diseases in years that were used to calculate damage costs.
4.4 Table 8: estimated average duration of diseases (undiscounted)
Disease | Duration (years) |
---|---|
Ischaemic Heart Disease (IHD) | 9.5 |
Asthma in Adults | 23.6 |
Asthma in Children | 36.2 |
Stroke | 14.8 |
Diabetes | 9.1 |
Lung cancer | 1.8 |
Hospital admissions are treated differently, as they’re single events rather than a year lived with a particular condition. Research conducted by Chilton and others (2004) also asked respondents about their willingness to pay to avoid hospitalisation. These values are used in damage cost pathways and rebased to 2017 prices. The values at 2022 prices are:
- £9,800 for a respiratory hospital admission
- £10,000 for a cardiovascular admission
4.5 Productivity
Productivity impacts of air pollution are valued following the approach in section 5 of Ricardo AEA (2014). This involves calculating the product of working time lost due to air pollution-related illness and an estimate of marginal productivity. Values for marginal productivity are taken from the Confederation of British Industry’s (CBI) ‘Workplace health and absence’ survey, which accounts for wage and non-wage costs.
4.6 Damage caused by sulphur dioxide to buildings
Damage to building materials covers:
- limestone
- sandstone
- mortar
- zinc used in galvanised steel
Quantification covers utilitarian buildings and infrastructure, but not cultural heritage. Response functions are based on 8 years of exposure of material specimens across Europe. These demonstrate SO2 to be the most harmful of the pollutants under conditions up to the mid-2000s, so analysis has focused on this pollutant.
Valuation is performed using repair cost data from the architecture and building sector. Repair is necessary once a critical loss of material (defined in relation to each material, based on how they are used) has occurred. Value is calculated via the change in frequency of repair operations.
A full account of the methods used is provided in the reports of the European Commission-funded ExternE Project. The value is estimated, on a national average basis, at £270 per tonne of SO2 emitted (2022 prices).
4.7 Damage caused by ozone to materials
Holland and others (1998) estimated that the effect of a population weighted 1ppb (parts per billion) change in ozone was £3.7m per annum (2005 prices). This relationship has been used in the damage cost estimates. The price base has been updated and conversion expressed in terms of population-weighted ozone concentration - parts per billion to microgram per cubic metre (1ppb to 2µg/m3). This gives the impact per tonne of NOx or VOC emitted via the ozone pathway in current prices.
4.8 Soiling of buildings due to PM
Rabl and others (1998) estimated the disamenity impacts of soiling due to PM by inferring the value from expenditure on renovation of buildings in France. The values from this research are used in the damage costs and equate to £642 (2022 prices) per tonne of PM10 emissions (and £995 per tonne when converted to emissions of PM2.5).
4.9 Ecosystem damages
Drawing on the Jones and others (2014) study, Table 7 details the valuation for different ecosystem pathways associated with key pollutants that are used to produce damage costs.
4.10 Total contribution of pathways to overall impact.
Following the IPA, damage costs are produced for more proportionate analysis where air quality impacts are less significant. Table 8 shows the breakdown of the damage cost for each pollutant by impact pathway. This also gives a sense of the most significant impacts in terms of value to society.
4.11 Table 9: central ecosystem pathway values (2022 prices)
An n/a entry in the table means no relevant impact or no impact assessed.
Ecosystem service (pathway) | NO2 (£/tonne) | NH3 (£/tonne) | SO2 (£/tonne) | O3* (£/ppb (7-month 24-hour mean)) | O3* (£/POD) |
---|---|---|---|---|---|
Provisioning services (Crop production) | n/a | n/a | n/a | n/a | -177,868,940 |
Provisioning services (Timber production) | -5 | -110 | n/a | n/a | n/a |
Provisioning services (Livestock production) | -10 | -334 | n/a | -1,295,793 | n/a |
Regulating services (CO2 GHG Emissions) | -251 | -5,892 | n/a | -26,688,465 | n/a |
Regulating services (N2O GHG Emissions) | 55 | 1,573 | n/a | n/a | n/a |
Regulating services (CH4 GHG Emissions) | n/a | n/a | -25 | n/a | n/a |
Cultural services (Recreational fishing) | 0 | 3 | n/a | n/a | n/a |
Cultural services (Biodiversity) | 121 | 487 | n/a | n/a | n/a |
*Jones and others (2014) present costs for decreases in NO2, NH3 and SO2 emissions and increases in O3 metrics. Defra have reversed the sign of the costs for O3 so that costs are shown for increases for all pollutants (negative numbers are benefits).
4.12 Table 10: low ecosystem pathway values (2022 prices)
An n/a entry in the table means no relevant impact or no impact assessed.
Ecosystem service (pathway) | NO2 (£/tonne) | NH3 (£/tonne) | SO2 (£/tonne) | O3* (£/ppb (7-month 24-hour mean)) | O3* (£/POD) |
---|---|---|---|---|---|
Provisioning services (Crop production) | n/a | n/a | n/a | n/a | -147,561,084 |
Provisioning services (Timber production) | -3 | -59 | n/a | n/a | n/a |
Provisioning services (Livestock production) | -6 | -212 | n/a | -526,454 | n/a |
Regulating services (CO2 GHG Emissions) | -106 | -2,489 | n/a | -17,975,193 | n/a |
Regulating services (N2O GHG Emissions) | 29 | 833 | n/a | n/a | n/a |
Regulating services (CH4 GHG Emissions) | n/a | n/a | -7 | n/a | n/a |
Cultural services (Recreational fishing) | 0 | 3 | n/a | n/a | n/a |
Cultural services (Biodiversity) | 39 | 164 | n/a | n/a | n/a |
*Jones and others (2014) present costs for decreases in NO2, NH3 and SO2 emissions and increases in O3 metrics. Defra have reversed the sign of the costs for O3 so that costs are shown for increases for all pollutants (negative numbers are benefits).
4.13 Table 11: high ecosystem pathway values (2022 prices)
An n/a entry in the table means no relevant impact or no impact assessed.
Ecosytem service (pathway) | NO2 (£/tonne) | NH3 (£/tonne) | SO2 (£/tonne) | O3* (£/ppb (7-month 24-hour mean)) | O3* (£/POD) |
---|---|---|---|---|---|
Provisioning services (Crop production) | n/a | n/a | n/a | n/a | - 177,868,940 |
Provisioning services (Timber production) | -5 | -110 | n/a | n/a | n/a |
Provisioning services (Livestock production) | -10 | -334 | n/a | -1,295,793 | n/a |
Regulating services (CO2 GHG Emissions) | -251 | -5,892 | n/a | -26,688,465 | n/a |
Regulating services (N2O GHG Emissions) | 55 | 1,573 | n/a | n/a | n/a |
Regulating services (CH4 GHG Emissions) | n/a | n/a | -25 | n/a | n/a |
Cultural services (Recreational fishing) | n/a | 3 | n/a | n/a | n/a |
Cultural services (Biodiversity) | 121 | 487 | n/a | n/a | n/a |
*Jones and others (2014) present costs for decreases in NO2, NH3 and SO2 emissions and increases in O3 metrics. Defra have reversed the sign of the costs for O3 so that costs are shown for increases for all pollutants (negative numbers are benefits).
4.14 Table 12: contribution of different impact pathways to overall national damage costs (central)
Impact pathway | NOx | SO2 | NH3 | VOC | PM2.5 |
---|---|---|---|---|---|
PM2.5 Chronic mortality | 1,565.00 | 9,320.70 | 5,274.90 | n/a | 42,391.60 |
PM2.5 Respiratory hospital admission | 9.5 | 56.9 | 32.2 | n/a | 258.6 |
SO2 Deaths brought forward | n/a | 39.5 | n/a | n/a | n/a |
SO2 Respiratory hospital admission | n/a | 85.8 | n/a | n/a | n/a |
O3 Deaths brought forward | -10.9 | n/a | n/a | 4.2 | n/a |
O3 Respiratory hospital admission | -62.5 | n/a | n/a | 24.3 | n/a |
NO2 Chronic mortality | 2,691.60 | n/a | n/a | n/a | n/a |
NO2 Respiratory hospital admission | 84.8 | n/a | n/a | n/a | n/a |
PM2.5 Productivity | 93.6 | 557.4 | 315.4 | n/a | 2,535.10 |
O3 Productivity | -63 | n/a | n/a | 24.5 | n/a |
O3 Material damage | -20 | n/a | n/a | 5.6 | n/a |
PM10 Building soiling | n/a | n/a | n/a | n/a | 994.2 |
SO2 Material damage | n/a | 269.5 | n/a | n/a | n/a |
O3 Ecosystems | -31.7 | n/a | n/a | 68.5 | n/a |
O3 Ecosystems | -72 | n/a | n/a | 45 | n/a |
NO2 Ecosystems | 120.9 | n/a | n/a | n/a | n/a |
NH3 Ecosystems | n/a | n/a | 486.8 | n/a | n/a |
PM2.5 IHD | 152.8 | 909.9 | 514.9 | n/a | 4,138.10 |
PM2.5 Stroke | 265.9 | 1,583.60 | 896.2 | n/a | 7,202.50 |
PM2.5 Lung Cancer | 20.4 | 121.4 | 68.7 | n/a | 552.1 |
PM2.5 Asthma (Children) | 616.4 | 3,671.20 | 2,077.70 | n/a | 16,696.90 |
NO2 Asthma (Small Children) | 2,079.00 | n/a | n/a | n/a | n/a |
NO2 Asthma (Older Children) | 707.6 | n/a | n/a | n/a | n/a |
Total damage cost (£/t in 2022 prices) | 8,148 | 16,616 | 9,667 | 172 | 74,769 |
4.15 Overlap of NOx and PM
Air pollutants are often emitted in mixtures. For example, road transport will typically produce emissions of both NOx and PM simultaneously. It can be difficult for epidemiological studies to isolate the health impacts of just one type of pollutant. Some measures will affect emissions of one type of pollutant more than others which we need to be aware of when using a method to estimate the damage associated with each pollutant individually.
Coefficients derived from studies that look at one pollutant only (‘single pollutant models’) may capture the effects of other pollutants and, therefore, be biased.
To correct this potential bias, COMEAP (page 54, section 7.5.1) recommend a reduction in the CRF associated with single pollutant NOx models:
“To assess pollution reduction measures which are specific to NOx/NO2 reduction, we have decided to recommend use of the summary estimate from a meta-analysis from the single pollutant models but we have used expert judgement to reduce this coefficient to account for confounding by both PM mass (in the light of the results of the two pollutant models) and for the possible effects of other pollutants which correlate more strongly with NO2 than PM. The majority view of the Committee is to recommend use of 25-55% (mid-point of range 40%) of the unadjusted coefficient 1.023 (95% CI: 1.008, 1.037) per 10 µg/m3 annual average NO2. This yields coefficients of 1.006 per 10 µg/m3 annual average NO2 and 1.013 per 10 µg/m3 annual average NO2 respectively.”
This 40% adjustment factor is applied to the CRF in the NO2 mortality associated with long-term exposure and NO2 morbidity pathways.
No adjustment factor is available for PM emissions. This means that the PM chronic mortality pathway does not account for the potential confounding effect of other correlated pollutants and is likely to be overestimated.
You should acknowledge this limitation and examine its potential effect on results through sensitivity analysis.
4.16 Conversion of PM10 to PM2.5
Damage costs for particulate matter are expressed in terms of emissions of PM2.5 (that is, particles of diameter 2.5µm or less). Some of the pathways included in those costs relate to PM10 (that is, particles of diameter 10µm or less). And for some activities it’s more common for emissions to be expressed in terms of PM10.
Typically, for any source of PM emissions, there will be a range of particle sizes. The National Atmospheric Emissions Inventory (NAEI) provides an estimate of emissions of both PM2.5 and PM10 for most sources. This allows you to:
- calculate the expected ratio of these emission categories for different sectors and emission sources
- convert estimates of PM10 emissions to estimates of PM2.5 emissions and to monetise the latter using damage costs
The damage costs that are applied to PM2.5 emissions contain all of the relevant impact pathways for PM10.
4.17 Sensitivities
Calculate impact pathways for low, central and high scenarios to capture uncertainties associated with the analysis. For NOx and PM, the scenarios differ in which impact pathways they incorporate.
Tables 1 to 3 show which impact pathways are incorporated into the sensitivities.
Table 13 shows the other variations that the low and high sensitivities take into account.
Tables 4, 5 and 6 the concentration response functions that are used in the low, central and high scenarios for each pathway.
4.18 Table 13: sensitivity scenarios
Variable | Pollutants affected | Low sensitivity scenario | Central sensitivity scenario | High sensitivity scenario |
---|---|---|---|---|
CRF sensitivity | All | Low CRFs (and large adjustment of NO2 chronic mortality effect) | Central CRFs (and central adjustment of NO2 chronic mortality effect) | High CRFs (and small adjustment of NO2 chronic mortality effect) |
Value of health impacts | All | Low impact values | Central impact values | High impact values |
Proportion of acute deaths valued at good health VOLY | NOx, SO2, VOC | 0% | 0% | 15% |
Life years lost for acute deaths | NOx, SO2, VOC | 2 months | 4 months | 6 months |
Cardiovascular hospital admission impact pathway included? | All | No | No | Yes |
Less certain morbidity pathways included? | All | No | No | Yes |
4.19 Overall uncertainty
You can capture the main identified uncertainties using the sensitivities and caveats covered in Table 9. But, there remains a large degree of uncertainty throughout the impact pathways process, including the:
- dispersion modelling that underpins the calculation of impacts – this will depend on the specific model employed
- potential impacts caused by exposure to air pollutants that have not yet been identified and quantified by the research community
- valuation of PM emissions have not been adjusted to account for potential confounding effects of other pollutants
5. Activity costs
Activity costs estimate the air quality impact of a change in fuel used in energy (electricity, gas, oil, coal, LPG, petroleum coke and biomass) and transport. So, when you do not know the changes in pollutant emissions, but do know the change in fuel, apply the activity damage costs (rather than the damage costs) to estimate the impact of air quality within a policy appraisal.
The activity costs are given in:
- p/kWh value for fuel for energy
- p/litre for the transport sector
Activity costs are presented at the national level but also broken down into various domestic classifications (for example inner conurbation, urban medium, rural).
The activity costs are calculated using the NAEI emission factors for NOx, PM2.5 and SO2 (emissions per kWh or litres of fuel used) with the appropriate damage costs (calculated through the impact pathway approach), for the geographical breakdown. The total costs for the 3 pollutants create the total fuel used activity cost.
The activity costs published by the Department for Business, Energy and Industrial Strategy (BEIS) as part of the Energy use and greenhouse gas emissions supplementary guidance to the Green Book. See Tables 14 and 15 in the Data Tables 1 to 19 excel workbook.