RIFE 29, Radioactivity in Food and the Environment, 2023
Updated 7 November 2024
Foreword
The UK’s environmental regulators and food safety agencies are pleased to present the 29th edition of the Radioactivity in Food and the Environment (RIFE) report.
Radiation and radioactive substances have had many beneficial uses, including their use in medical diagnostics and therapies and in power generation. They are unlikely to be harmful if controlled in the right way. Regulation aims to ensure these benefits, whilst keeping people and the environment safe. This regulation is embedded into relevant legislation, such as the Environmental Authorisations (Scotland) Regulations 2018, Environmental Permitting Regulations (England and Wales) 2016 the Radioactive Substances (Modification of Enactments) Regulations (Northern Ireland) 2018. Our combined independent monitoring of radioactivity in food and the environment is an important part of our regulatory process. This also fulfils a vital role in providing reassurance to members of the public.
In common with previous issues of this report, RIFE 29 sets out the findings of the monitoring programmes of radioactivity in food and the environment carried out in 2023 throughout the UK. These monitoring programmes are undertaken by the Environment Agency, Food Standards Agency, Food Standards Scotland, Natural Resources Wales, Northern Ireland Environment Agency, and the Scottish Environment Protection Agency.
The monitoring results and subsequent assessments presented in this report demonstrate that radioactivity in food and the environment is safe. The exposure of members of the public to radiation, resulting from authorised discharges of radioactive waste and direct radiation, near nuclear and non-nuclear sites was far below the legal limit (1mSv) in 2023.
The RIFE monitoring programme supports the requirements of the permitting legislation across the UK, together with other national and international agreements, policies, regulations and standards.
This report was compiled by the Centre for Environment, Fisheries and Aquaculture Science on behalf of the Environment Agency, Food Standards Agency, Food Standards Scotland, Natural Resources Wales, Northern Ireland Environment Agency and the Scottish Environment Protection Agency.
Requests for supporting documents and other information should be addressed to:
- in England and Wales, Radiological Monitoring and Assessment Team of the Environment Agency ([email protected]), Food Policy Division of the Food Standards Agency ([email protected]) or Natural Resources Wales ([email protected])
- in Scotland, the Radioactive Substances Unit of SEPA ([email protected]) or Food Standards Scotland ([email protected])
- in Northern Ireland, the Industrial Pollution and Radiochemical Inspectorate of NIEA ([email protected])
Or alternatively,
Environment Agency
Ghyll Mount
Gillian Way
Penrith 40 Business Park
Penrith
Cumbria
CA11 9BP
Food Standards Agency
Food Policy Division
Clive House
70 Petty France
London
SW1H 9EX
Food Standards Scotland
4th Floor
Pilgrim House
Old Ford Road
Aberdeen
AB11 5RL
Cyfoeth Naturoil Cymru / Natural Resources Wales
Welsh Government Offices
Cathays Park
King Edward VII Avenue
Cardiff
CE10 3NQ
Northern Ireland Environment Agency
Industrial Pollution and Radiochemical Inspectorate
Klondyke Building
Cromac Avenue
Lower Ormeau Road
Belfast
BT7 2JA
Scottish Environment Protection Agency
Radioactive Substances Unit
Angus Smith Building
6 Parklands Avenue
Eurocentral
Holytown
North Lanarkshire
ML1 4WQ
Technical summary
This section is divided into the following topics to highlight the scope of this report. These are:
-
radiation exposures (doses) to people living near UK nuclear licensed sites
-
radioactivity concentrations in samples collected near UK nuclear licensed sites
-
external dose rates measured near UK nuclear licensed sites
-
UK nuclear licensed site incidents and non-routine surveys
-
habits surveys near UK nuclear licensed sites
-
monitoring of radioactivity at locations remote from UK nuclear licensed sites (overseas incidents, non-nuclear sites and regional monitoring across the UK)
-
the environmental radioactivity monitoring programmes
Radiation exposure (doses) to people living near UK nuclear licensed sites
Radiation doses to people living near nuclear licensed sites are assessed using data from radiological monitoring programmes, which cover both food and the environment. Radionuclide concentrations, dose rates, and information on the habits of people living near the sites are used to estimate doses. Where monitoring data are not available, some environmental concentrations are estimated by environmental transfer modelling of reported discharges. People’s exposure to radiation (doses) can vary from year to year, due to changes in radionuclide concentrations and external dose rates. Changes in habits data and information, in particular food consumption, can also cause the estimates of dose to vary.
The dose quantity presented in this summary is known as the ‘total dose’. This is made up of contributions from all sources of radioactivity from man-made processes. Source specific dose assessments are also carried out in some cases to provide additional information and to compare with the ‘total dose’ assessment method.
Figure S, shows the assessed ‘total doses’ in 2023 (as bars) across the UK, ranging from less than 0.005mSv to 0.23mSv at Sellafield in comparison to the dose limit to members of the public, the blue bar. Table S2 (main RIFE page ) contains the numerical values of the assessed ‘total doses’ presented in Figure S and includes the major contributors to dose. These ‘total doses’ are due to the combined effects of authorised/permitted waste discharges and direct exposure from the site (‘direct radiation’) on those people most exposed to radiation near all major nuclear licensed sites in the UK.
Figure S. ‘Total doses’ in the UK due to radioactive waste discharges and direct radiation, 2023 (Exposures at Sellafield, Whitehaven and Drigg receive a significant contribution to the dose from technologically enhanced naturally occurring radionuclides from previous non-nuclear industrial operations). Small doses less than or equal to 0.005mSv are recorded as being 0.005mSv.
Site | Dose, mSv |
---|---|
Sellafield | 0.23 |
Whitehaven and LLWR near Drigg | 0.23 |
Capenhurst | 0.15 |
Amersham | 0.089 |
Winfrith | 0.054 |
Barrow | 0.042 |
Springfields | 0.040 |
Hinkley | 0.032 |
Dounreay | 0.028 |
Hartlepool | 0.015 |
Heysham | 0.015 |
Hunterston | 0.013 |
Aldermaston and Burghfield | 0.011 |
Chapelcross | 0.010 |
Sizewell | 0.010 |
Rosyth | 0.008 |
Torness | 0.007 |
Trawsfynydd | 0.007 |
Derby | 0.006 |
Berkeley and Oldbury | 0.005 |
Bradwell | 0.005 |
Devonport | 0.005 |
Dungeness | 0.005 |
Faslane | 0.005 |
Harwell | 0.005 |
Wylfa | 0.005 |
Dose limit | 1 |
Doses to individuals are determined for those people most exposed to radiation (‘representative person’[footnote 1]). The estimated doses are compared with legal limits for the public. The method used to calculate doses to each hypothetical individual is based on guidance from the National Dose Assessment Working Group (NDAWG). NDAWG guidance proposes developing a series of habits profiles of people living and consuming food grown (or sourced) near nuclear licensed sites. These are derived from the habits survey data. Each habits profile provides information on their respective food consumption and occupancy rates. Doses for each habits profile are calculated and the ‘representative person’ is that profile which receives the highest dose.
In 2023, radiation doses from authorised/permitted releases of radioactivity to people living around nuclear licensed sites, remained well below the UK national limit of 1 millisievert (mSv, a measure of dose) per year (see Appendix 2 for explanation of dose units).
The locations where the public received the highest doses in 2023 were similar to those in 2022. These were the Cumbrian coastal community[footnote 2] near Sellafield (0.23mSv), Capenhurst (0.15mSv) and Amersham (0.089mSv). The doses received near Capenhurst and Amersham were dominated by direct radiation from sources on the sites.
The highest dose to the Cumbrian coastal community near Sellafield was mostly due to historical liquid discharges. In 2023, the representative person from the Cumbrian coastal community, was a high-rate crustacean consumer (who also consumed significant quantities of other seafood) and unchanged from that in 2022. The estimated dose was 0.23mSv in 2023. Most of this dose (0.21mSv) was due to the historical discharges of technologically enhanced naturally occurring radioactive material (TENORM) from the former phosphate processing plant near Whitehaven. The remainder of the dose (0.019mSv) was due to the permitted discharges of artificial radionuclides by the nuclear industry and includes a contribution from sources of direct radiation on the Low-Level Waste Repository (LLWR) site near Drigg. In the previous year (for 2022), the representative person received a dose of 0.24mSv (including a contribution of 0.22mSv and 0.014mSv related to the former phosphate processing plant and the nuclear industry, respectively). The decrease in ‘total dose’ was mostly attributed to the revision of habits information particularly the reduced consumption rate of both fish and crustacean species. The largest contribution to dose to seafood consumers in the Cumbrian coastal community was from the radionuclide polonium-210.
Polonium-210 is a significant contributor of the dose to the most exposed members of the public because it has a relatively high dose coefficient (a factor used to convert an intake of radioactivity into a radiation dose) as recommended by the ICRP. Polonium-210 is present in the environment from natural sources and from TENORM which used to be discharged from the former phosphate processing plant. Nevertheless, polonium-210 concentrations in crustacean samples continued to be within or close to the expected range due to natural sources in 2023. The discharge effects from the Sellafield site and the former phosphate processing plant (near Whitehaven) both impact the same area and therefore the contributions to doses from both sources are considered in Section 3.3.1.
In Scotland, the representative person consuming food (fish, shellfish and wildfowl) collected from areas along the Dumfries and Galloway coastline received the highest source specific dose[footnote 3] from authorised releases of radioactivity. The dose to adults was 0.021mSv in 2023, a decrease from 2022 (0.024mSv). As in previous years, most of the dose in 2023 was due to the effects of historical discharges from the Sellafield site.
The highest dose in Wales was near the Trawsfynydd nuclear power station. This site is being decommissioned. The representative person was a consumer of locally produced foodstuffs, and the dose was primarily due to historical permitted discharges from the site. The source specific dose[footnote 3] to 1-year-old infants was 0.034mSv in 2023; a slight decrease from 2022 (0.038mSv).
Radioactivity concentrations in samples collected near UK nuclear licensed sites
There were no major variations in environmental concentrations of radioactivity in 2023 compared to those in 2022. Near Sellafield, the environmental concentrations of most radionuclides have declined over the past 3 decades, albeit much more slowly in recent years, due mainly to strict regulation. In 2023, mean concentrations of plutonium-239+240 and americium-241 in winkles (Nethertown) and lobster (Sellafield Coastal Area) in 2023 were the lowest reported values in recent years.
In 2018, a review of the 2009 UK Radioactive Discharge strategy was published (Department for Business Energy and Industrial Strategy 2018). The review demonstrates clear evidence of progress being made by the UK in meeting the outcomes of the 2009 strategy and contributing towards the objectives of the OSPAR[footnote 4] radioactive substances strategy (RSS). Specifically, significant progress has been made towards achieving progressive and substantial reductions in radioactive discharges. Progress is also being made to achieving progressive reductions in concentrations of radionuclides in the marine environment and in achieving progressive reductions in human exposures to ionising radiation (see appendix 2), as a result of planned reductions in discharges (OSPAR 2022).
The OSPAR Radioactive Substances Committee published its Fifth Periodic Evaluation in 2022, which demonstrated that Contracting Parties, including the UK, have successfully fulfilled the objectives of the OSPAR RSS for 2020 under the North-East Atlantic Environment Strategy (NEAES) 2010 – 2020 and have made significant progress against the ultimate aim of radionuclide concentrations in the environment near background values for naturally occurring radionuclides and close to zero for artificial radionuclides (see Section 1.3.2 for further details) (OSPAR 2022). The UK specific outcomes in the fifth periodic evaluation (see Annex 2, (OSPAR 2022)), reinforces the findings of the 2018 review of the 2009 UK strategy for radioactive discharges.
External dose rates measured near UK nuclear licensed sites
Radioactivity in sediments in intertidal areas can potentially make a contribution to the total radiation exposure to members of the public. For this reason, in situ measurements of radiation dose rates are taken over exposed areas of sediment. These ‘external doses’ are included in the assessment of doses to the public where they are higher than natural background rates. To determine the dose to the public from any radioactivity that may be present as a result of authorised/permitted discharges, natural background rates are subtracted from the measured dose rates in the assessment.
There were no major changes in external dose rates in intertidal areas in 2023 compared with 2022. At most locations, the external dose rates were close to background rates. Rates were higher in some estuaries near Sellafield (up to twice the background rate) and in the Ribble Estuary.
UK nuclear licensed sites incidents and non-routine surveys
During 2023, as a result of an ongoing programme of monitoring by the operator, radioactive items (particles and objects[footnote 5]) from Sellafield were detected on Cumbrian beaches and removed (81 in the 2023 calendar year). The advice from the UK Health Security Agency (UKHSA) and the Food Standards Agency (FSA) is that the risk to the public from the radioactive particles and larger objects found on West Cumbrian beaches is very low. A programme of work is in place to meet the primary aim of providing reassurance that overall risks to beach users remain at, or below those estimated in the UKHSA risk assessment. UKHSA published a summary report of assessing the risk to people’s health from radioactive objects on beaches around the Sellafield site in February 2020 (Oatway, Cabianca & Jones 2020).
At Dounreay, the comprehensive beach monitoring programme continued for fragments of irradiated nuclear fuel (particles). Last year, the number of particles recovered and the range in radioactivity content were similar to that observed in recent years. Fishing restrictions in a specific area around Dounreay are still in force under the Food and Environment Protection Act (FEPA) 1985 (United Kingdom - Parliament 1985).
Special (or ‘ad hoc’) sampling related to nuclear licensed site operation is carried out at sites when needed or to provide one-off data sets. No such need arose in 2023.
Habits surveys near UK nuclear licensed sites
For ‘total dose’ assessments, habits data are used to define the exposure pathways (such as eating locally produced food and time spent on beaches) for members of the public. Habits data are used to define one or more hypothetical individuals[footnote 6] (for each pathway). The doses to each hypothetical individual are calculated and the individual with the highest dose is the representative person. The dose calculated in this way is considered representative of the dose to the most highly exposed individuals in the population.
In 2023, the regular programmes of habits surveys continued using existing methods. Surveys were carried out at the LLWR near Drigg, and Sellafield in England, at Faslane and Torness in Scotland, and Wylfa in Wales.
These habits surveys give site-specific information on the diet and occupancy habits of people near nuclear licensed sites. The findings were used to confirm the adequacy of current monitoring programmes, to strengthen and update them with a better representation of relevant exposure pathways, and to improve the assessment of doses to members of the public near nuclear licensed sites.
Monitoring of radioactivity at locations remote from UK nuclear licensed sites
Additional monitoring in the UK and surrounding seas was carried out to assess the impact of (1) non-nuclear sites, (2) the concentrations of radioactivity across the UK (measured as part of the regional monitoring programme) and (3) overseas incidents that may have introduced radioactivity into the environment.
1) Non-nuclear sites
In the past, liquid waste slurry (regarded as TENORM) containing thorium and uranium was discharged from a phosphate processing plant near Whitehaven (Cumbria) into the Irish Sea. These discharges have resulted in an increase in the concentrations of naturally occurring radionuclides in the environment through the production of radioactive decay products (from the radioactive decay of radionuclides previously discharged to sea).
Historically, the concentrations of two decay products, polonium-210 and lead-210, in fish and shellfish (near Whitehaven) have been found to be higher than the maximum expected concentration ranges due to naturally occurring radioactivity. Concentrations have declined significantly since the plant ceased operations in 1992. Since then, polonium-210 and lead-210 have been within or close to the expected natural background concentration ranges. Estimates of the enhanced concentrations in seafood are made by subtracting the median of the expected natural concentration range of these radionuclides from the measured values. These radionuclides are important in that small changes in values above background, significantly influence their contribution to the combined dose. The representative person in the area who consumed large amounts of seafood received a dose of 0.21mSv in 2023, and polonium-210 was the most contributing radionuclide. This estimation of dose also includes a much smaller contribution from the effects of discharges from the nearby nuclear site at Sellafield.
Concentrations of tritium were found in leachate from some landfill sites, at quantities that were of very low radiological significance. There are several disposal routes for radioactive waste to landfill that could contain tritium, for example, from hospitals and industrial sites or due to disposals of gaseous tritium light devices (such as fire exit signs).
Work to address radioactive contamination is ongoing at Dalgety Bay, Fife. Public protection measures have been established and these were maintained during 2023 and into 2024. This includes continuing a monthly beach monitoring and particle recovery programme. The FEPA Order issued by Food Standards Scotland (FSS) (then FSA in Scotland), prohibiting the collection of seafood from the Dalgety Bay area, remains in force. The remediation contract was awarded by the MOD in February 2020 and an Environmental Authorisations (Scotland) Regulations (EASR18) permit for the required work was granted in May 2021. Remediation work took place between 2021 and 2023. The area will now undergo a period of evaluation to ensure that the remedial works have been successful.
Further details can be found in Section 7.6 of this report and on the radioactive substances pages of Scottish Environmental Protection Agency (SEPA’s) website: SEPA Dalgety Bay.
2) Regional monitoring of radioactivity across the UK
Regional monitoring in areas remote from nuclear licensed sites continued in 2023 to:
- establish the extent of long-distance transport of radioactivity from UK and other nuclear licensed sites
- identify any general contamination of the food supply and the environment
- provide data in compliance with UK obligations under the OSPAR Convention
From the monitoring of artificial radioactivity in Northern Ireland, consumer doses were estimated to be less than 1% of the annual limit of 1mSv for members of the public in 2023. A survey on the Channel Islands confirmed that doses due to discharges from the French reprocessing plant at La Hague and other local sources were less than 0.5% of the legal limit.
Food and sources of public drinking water that make up a general diet for people were analysed for radioactivity across the UK. In 2023, artificial radionuclides only contributed a small proportion of the total public radiation dose in people’s general diet, and this was much less than 0.5% of the legal limit.
The distribution of radionuclides in coastal seas continues to be monitored away from nuclear licensed sites. This supports the UK’s marine environmental policies and international treaty commitments. Government research vessels are used in the sampling programme and the results have been used to show trends in the quality of the UK’s coastal seas. These surveys, together with the results of monitoring at nuclear licensed sites, contribute to the UK data submitted to the OSPAR Commission. These data also help measure progress towards the UK government and devolved administrations objectives for improving the state of the marine environment. Disposal of dredged material from harbours and other areas in England is licensed under the Marine and Coastal Access Act 2009 (MCAA) (United Kingdom - Parliament 2009). In 2023, no requests were received by the Marine Management Organisation (MMO) to apply for additional licences for the disposal of dredged material (containing radioactivity) at sea.
3) Overseas incidents
The accident at the Fukushima Dai-ichi nuclear power station in Japan in March 2011 resulted in significant quantities of radioactivity being released into the air and sea. Controls on imported food and animal feed products from Japan to the UK mainland were revoked in June 2022, see previous RIFE reports for more details. In Northern Ireland, European Regulations continue to apply under the terms of the UK’s withdrawal agreement from the EU.
Some food imported into the UK may contain radioactive contamination from the 1986 Chernobyl accident and other known or unknown sources. A monitoring system is in place to detect radioactivity in consignments. In 2023, no significant radioactivity (above screening levels) was detected at entry points and there was no need to introduce food safety controls on any consignments.
The environmental radioactivity monitoring programmes
The environmental monitoring programmes in this report are carried out on behalf of the Environment Agency, FSA, FSS, Natural Resources Wales (NRW), Northern Ireland Environment Agency (NIEA) and SEPA and are independent of the industries discharging radioactive wastes. The programmes include monitoring in support of the Scottish Government, Channel Island states, Department of Agriculture Environment and Rural Affairs (DAERA), Department for Energy Security & Net Zero (DESNZ), Department for Environment, Food & Rural Affairs (Defra), NRW and the Welsh Government. The monitoring programmes involve specialist laboratories working together, each with rigorous quality assurance procedures, and a wide range of sample collectors throughout the UK.
Overall, around 10,000 analyses and dose rate measurements were completed in 2023. The analytical results of the environmental radioactivity monitoring programmes are reported in tables in the relevant sections (Sections 3 to 8). The values provided in the tables are given in 3 different forms,
- measurable values (referred to as ‘positively detected’)
- less than values (that is, the lowest activity concentration, or dose rate measurement, that can be reliably detected for a given analytical method)
- not detected (ND) values (meaning that insufficient evidence is available to determine the existence of a radionuclide)
Where the results are an average of more than one measurement, and each value is positively detected, then the result in the table is reported as being positively detected. Alternatively, where there is a mixture of values (both positively detected and less than values), or all are less than values, then the result in the table is reported as a less than value, preceded by a ‘less than‘ symbol (<). The Environment Agency has produced a document that includes radionuclide information, such as the sources, uses, modes of release and nuclear decay data (Environment Agency 2003).
Only results that are the most relevant for assessing the impact of radionuclide concentrations in food and the environment are provided in each site table. This ensures that reporting of the more meaningful results is manageable. For example, gamma-ray spectrometry can provide a large number of less than values and may not be reported. To identify the most relevant values, to be included in each individual table, one or more of the following conditions is required:
- all radionuclide results (both positively detected and less than values) are reported in the site table if the radionuclide is specified in the relevant permit/authorisation (as indicated for each site in Appendix 1, Table A1.1 and Table A1.2)
- all radionuclide results (both positively detected and less than values) are reported that have been analysed using a radiochemistry method (for example, plutonium radionuclides)
- for any radionuclide that is reported as positively detected in the previous 5 years of annual reporting, all activity concentration data of that radionuclide are reported (they are only excluded from the table after 5 continuous years of reporting ‘less than values’)
- for any radionuclide that is reported as positively detected in one of the samples, all activity concentration data of that radionuclide are reported for other samples presented in the table (terrestrial and marine) in that year
- naturally occurring radionuclides measured by gamma-ray spectrometry (for example potassium-40) are not usually reported unless the intention is to establish whether there is any enhancement above the expected background concentrations (for example from landfill sites)
More information about programmes described in this report is available from the sponsoring agencies. Their contact details can be found at the end of the Foreword of this report. The results of the analysis of food samples collected near nuclear licensed sites in England and Wales are published on the FSA’s website (main FSA webpage).
1 Introduction
Overview
- The Radioactivity in Food and the Environment (RIFE) report represents the collaboration between the Environment Agency, Natural Resources Wales (NRW), Northern Ireland Environment Agency (NIEA) and Scottish Environmental Protection Agency (SEPA) referred to together as the environment agencies in this report, Food Standards Agency (FSA) and Food Standards Scotland (FSS). The monitoring programme is independent of the nuclear industry.
- RIFE provides a transparent check on food safety and the public’s exposure to radiation according to the Ionising Radiation (Basic Safety Standards) (Miscellaneous Provisions) (Amendment) (EU Exit) Regulations 2018 and the Environmental Permitting Regulations 2019 (as amended), retained from the EU Basic Safety Standards Directive 2013 (BSSD 13).
- The monitoring programme results support the UK in meeting its international treaty obligations.
- Annual radiation doses are summarised for major industrial sites; all doses were below the legal limit of 1mSv in 2023.
This section:
- describes the scope and purpose of the UK monitoring programmes for RIFE
- provides a summary of the key results in terms of radiation exposures at each major industrial site in 2023
- gives an overview of the main sources of radiation in a regulatory context
1.1 Scope and purpose of the monitoring programmes
In England and Wales, the FSA and the Environment Agency[footnote 7] carry out food and non-food (including seawater, sediments, dose rate) monitoring, respectively. SEPA (working closely with FSS on its programme) and the NIEA both undertake food and non-food monitoring in Scotland and Northern Ireland, respectively. Food monitoring includes the collection and analysis of cow’s milk (unless otherwise specified in this report). Surveillance of imports through points of entry continued in 2023. The regular national programme of monitoring of drinking water, air and rain continued on behalf of the DESNZ, NIEA and the Scottish Government. The FSA and SEPA (as part of the joint SEPA/FSS monitoring programme) also carry out UK monitoring of milk and canteen meals that are collected remotely from nuclear licensed sites. Annual surveys of seas around the UK (including locations away from nuclear licensed sites) are monitored on behalf of DESNZ.
The FSA has responsibility for food safety in England, Northern Ireland, and Wales, and FSS has responsibility in Scotland. The environment agencies are responsible for regulating environmental protection in England, Northern Ireland, Wales, and Scotland, respectively. This includes the regulation of radioactive discharges and radioactive waste disposal from nuclear and non-nuclear sites.
The current UK legislation, relating to radioactivity, provides uniform safety standards to protect the health of workers and members of the public. These basic safety standards are retained from European Council (EC) Directives, the most recent one being the Basic Safety Standards Directive 2013 or ‘BSSD 13’ (European Commission 2014). This lays down basic safety standards for protecting people against the dangers arising from exposure to ionising radiation. The RIFE report and the associated monitoring programmes were designed to conform to the requirements of Article 36 of the Euratom Treaty (see Section 8 and Appendix 1 in previous RIFE reports, for more details). Specifically, it provides estimates of annual doses to members of the public from authorised practices and enables these results to be made available to stakeholders. Following its withdrawal from the Euratom Treaty, the UK is no longer required to report these data to the EC and has agreed a Nuclear Cooperation Agreement (NCA) with the EU, ensuring both parties continue working together on civil nuclear matters including safeguards, safety, and security. In late December 2020, DESNZ published its transboundary directions to the environment agencies, available from DESNZ Transboundary directions. These directions replace the requirements of Article 37 (related to the transboundary radiological impact of releases during normal operation) of the Euratom treaty following the UK withdrawal from the Euratom Treaty.
The Ionising Radiation (Basic Safety Standards) (Miscellaneous Provisions) (Amendment) (EU Exit) Regulations 2018 (UK Statutory Instruments 2018a) came into force to transpose parts of BSSD 13, not already covered within existing statutory regimes. These regulations impose duties on appropriate ministers to ensure that certain functions are carried out in relation to exposures from contaminated land, exposures from buildings or contaminated commodities and to raise awareness and issue guidance about orphan sources (which are not under regulatory control but pose a radiological hazard – see Appendix 2 for definition).
The requirements for regulating public exposure from the disposal of radioactive waste in England and Wales are set out in the Environmental Permitting (England and Wales) Regulations 2016 (EPR 16) (United Kingdom - Parliament 2016), in particular Schedule 23 ‘radioactive substances activities’. These regulations were amended in 2018 by the Environmental Permitting (England and Wales) (Amendment) (No. 2) Regulations 2018 (EPR 18) (United Kingdom - Parliament 2018) in order to transpose changes brought about by BSSD 13, and then by the Environmental Permitting (England and Wales) (Amendment) (EU Exit) Regulations 2019 (EPR 19) in 2019 (United Kingdom - Parliament 2020a). This was to ensure that the regulations remain fully operable at the end of the transition period following the UK’s exit from the EU. Further changes were made in the Waste and Environmental Permitting etc. (Legislative Functions and Amendment etc.) (EU Exit) Regulations 2020, which transferred some functions from the European Commission to the Secretary of State and the devolved administrations (United Kingdom - Parliament 2020b).
In 2018, the Radioactive Substances (Modification of Enactments) Regulations (Northern Ireland) 2018 (RSR 18) came into force for radioactive substances activities in Northern Ireland (Statutory Rules of Northern Ireland 2018) by amending the Radioactive Substances Act 1993 (RSA 93) (United Kingdom - Parliament 1993). A guidance document was also published in 2018, providing the scope of and exceptions from the radioactive substances legislation in England, Wales, and Northern Ireland (Department for Business Energy & Industrial Strategy and others, 2018).
The requirements for regulating public exposure from the disposal of radioactive waste in Scotland is set out in the Environmental Authorisations (Scotland) Regulations 2018 (EASR18) (Scottish Government 2018), in particular Schedule 8 ‘radioactive substances activities’. EASR18 currently applies to both offshore and onshore activities in Scotland. A guidance document has also been published to support the implementation of the regulations. There are 4 types of authorisation under EASR18: general binding rules, notification, registration and permit (more information can be found at: SEPA environmental authorisations). The new regulations aim to provide an integrated authorisation framework, which will bring together as far as possible, the authorisation, procedural and enforcement arrangements relating to water, waste management, radioactive substances and pollution prevention and control. This framework is being developed in a phased manner and currently the regulations only apply to radioactive substance activities. In late 2022, SEPA initiated a consultation on the implementation of the EASR18 framework before developing proposals to incorporate the regulation of other environmental activities under EASR18. The consultation closed in March 2023, with the majority of responses supportive of SEPA’s implementation of the EASR18 framework.
In order to transpose the requirements of BSSD 13, the Ionising Radiations Regulations 2017 (IRR 17) (United Kingdom - Parliament 1999a) came into force in 2018 (replacing the Ionising Radiations Regulations 1999). The Health and Safety Executive (HSE) has also provided practical advice (Code of Practice) to help operators comply with their duties under IRR 17 (Health and Safety Executive 2018). IRR 17 controls the radiation exposure of workers and the public apart from that resulting from the permitted disposal of radioactive waste, which is regulated by the environment agencies under the various permitting legislation described previously. The Ionising Radiation (Basic Safety Standards) (Miscellaneous Provisions) Regulations 2018 (UK Statutory Instruments 2018b) transposes Directive 2013/59/EU to ensure the UK is committed to maintaining high safety standards for protection against exposure to ionising radiation and includes updated scientific methods (for example, analytical methods or abatement techniques).
The Environment Agency and SEPA also have broader responsibilities under the Environment Act 1995 (United Kingdom - Parliament 1995a) for environmental protection including determining general concentrations of pollution in the environment. The responsibilities for Wales under the Environment Act 1995, have been transferred to NRW.
The monitoring programmes have several purposes:
- environmental and food results are used to estimate and assess dose to the public to confirm that the controls and conditions placed in the authorisations/permits provide the necessary protection and to ensure compliance with legal dose limits
- ongoing monitoring helps to establish the long-term trends in concentrations of radioactivity over time near, and at distance from, nuclear licensed sites
- the results are also used to confirm the safety of the food chain
- monitoring the environment provides indicators of radionuclide dispersion around each nuclear site
Most of the monitoring carried out and presented in this report concerns the local effects of discharges from nuclear licensed sites in the UK. Monitoring of food and the environment away from nuclear licensed sites is also carried out, giving information on background concentrations of radionuclides. In previous years, the Environment Agency (with support from NRW), the FSA, FSS and SEPA have all completed reviews of their environmental radioactivity monitoring programmes. Further information is available in earlier RIFE reports (for example, (Environment Agency and others, 2020)). Reviews are carried out to ensure the monitoring programmes are appropriate and are consistent with advice in the agency technical guidance (Environment Agency, Food Standards Agency & Scottish Environment Protection Agency 2010; Scottish Environment Protection Agency 2019a), resulting in an adjustment and consolidation of the monitoring around some sites. Year on year, the monitoring programmes are also affected by sample availability.
The Environment Agency, FSA, FSS, NRW, NIEA, SEPA and DESNZ have produced a RIFE summary. This summary report was combined with the UK report on the application of Best Available Techniques (BAT) in civil nuclear facilities (2017 to 2021), which was submitted to the Radioactive Substances Committee of the OSPAR Commission as the UK statement on the implementation of OSPAR Recommendation 2018/1 on Radioactive Substances in early 2023 and is available at the following webpage - UK implementation reporting on the OSPAR website.
The analysis and measurements for the monitoring programmes was carried out by various UK laboratories, including those listed below. These laboratories also carried out most of the sample collection for the programmes:
- Centre for Environment, Fisheries and Aquaculture Science (Cefas)
- UK Health Security Agency (UKHSA)
- SOCOTEC UK Limited
Details of the methods of sampling and analysis are presented in Section 2 of this report. Section 2 also explains how results are interpreted in terms of public radiation exposures. A summary of the assessment approach and current trends in doses is given in Section 1.2.
1.2 Summary of radiation doses
1.2.1 The assessment process
Most of the monitoring was carried out to determine the effects of discharges from nuclear and non-nuclear operations on the food people consume and their environment. The results are used to estimate and assess annual radiation doses to the public that can then be compared with the relevant dose limits. Dose assessments are retrospective in that they apply to 2023 using monitoring results for that year. The radioactivity concentrations and dose rates reported include the combined radiological impact of all discharges, up to the time of sampling.
In this report, 2 main types of retrospective doses are assessed. The sources considered, habits and monitoring data used, and the dose calculations are outlined in the sections below. The primary purpose of each assessment type is to assess the main sources of exposure at each site for comparison with the 1mSv limit. The first type of assessment considers the doses from radioactive discharges (gaseous and liquid) to the environment from nuclear licensed sites combined with the dose from site radiation sources (direct radiation). This assessment gives an estimate of the annual ‘total dose’ to people living near the nuclear licensed sites. The ‘total dose’ assessment is the main method for estimating radiation exposure to the public.
Type of assessments and dose quantity
- ‘Total dose’ - ‘total dose’
- Source specific dose – terrestrial - dose from gaseous discharges
- Source specific dose – aquatic - dose from liquid discharges
- Source specific dose – direct radiation (dose estimates provided by the ONR) - dose from direct radiation
Sources considered
Total Dose
- Gaseous discharges, liquid discharges, and sources of direct radiation on site
Source specific dose – terrestrial
- Gaseous discharges
Source specific dose – aquatic
- Liquid discharges
Habits data, for example, food consumption rates or occupancy of beaches
Total dose
- Define usage of pathways relating to all sources at site
Source specific dose – terrestrial
- Define usage of pathways relating to gaseous discharges at site
Source specific dose – aquatic
- Define usage of pathways relating to liquid discharges at site
Monitoring data
Total dose
- Collate monitoring data for relevant pathways, for example, radionuclide concentrations in food or dose rates on beaches. At Sellafield and LLWR near Drigg, food chain modelling is used for limited radionuclides. At the majority of sites, PC CREAM 08 is used to calculate the external and inhalation doses from gaseous discharges. Doses to sewage workers from liquid discharges are calculated using the Environment Agency’s IRAT model. Simple models are used to calculated external dose rates from activity concentrations in sediment
Source specific dose – terrestrial
- Collate monitoring data for relevant pathways, for example, radionuclide concentrations in food. At Sellafield and LLWR near Drigg, food chain modelling is used for limited radionuclides. At the majority of sites, PC CREAM 08 is used to calculate the external and inhalation doses from gaseous discharges
Source specific dose – aquatic
- Collate monitoring data for relevant pathways, for example, radionuclide concentrations in food or dose rates on beaches. Doses to sewage workers from liquid discharges are calculated using the Environment Agency’s IRAT model. Simple models are used to calculated external dose rates from activity concentrations in sediment
Dose calculations
Total dose
- Calculate dose from all sources to individuals who may represent those most exposed. Select the highest dose for the person representing the most exposed
Source specific dose – terrestrial
- Calculate dose from gaseous discharges to people representing those most exposed
Source specific dose – aquatic
- Calculate dose from liquid discharges to people representing those most exposed
Exposure from direct radiation may be a significant contributor to dose, close to a nuclear site, due to radiation emitting from sources on the site[footnote 8]. The Office for Nuclear Regulation (ONR) is responsible for regulating direct radiation. In 2018, Électricité de France (EDF) Energy revised its method of direct dose assessment (for the calendar year) for operating power stations based on measurements of external radiation dose rates at the site boundary, distances to the point of exposure and occupancy data (EDF Energy 2018). This is different to the previous method based on generic arguments considering the low dose rates from Advanced Gas-cooled Reactor (AGR) and Pressurised Water Reactor (PWR) power stations. Therefore, values since 2018 will differ from the generic values given previously. The operators of nuclear licensed sites provide estimates of direct radiation doses to the ONR (Table 1.1); annual exposure data are then made available for use in ‘total dose’ assessments. These dose assessments use recent habits survey data which have been profiled using an agreed method (Camplin, Grzechnik & Smedley 2005). Data tables and the detailed results of the dose assessments, in Open Document Spreadsheet (ODS) format, are downloadable from themain RIFE page.
The second type of assessment estimates annual dose from specific sources and associated exposure pathways (see Appendix 2, and Appendix 5 for more information). These dose assessments provide reassurance on the robustness and reasonableness of the annual ‘total dose’ method (which is the preferred assessment type (Environment Agency, Scottish Environment Protection Agency, and others, 2012)) and provide information for a range of additional exposure pathways. The sum of the doses from specific sources (terrestrial and aquatic) cannot be directly compared to the assessment of ‘total dose’ from all sources. This is because the assessment methods use different ways of defining the most exposed people.
Both types of assessment consider those people in the population most exposed to radiation (the ‘representative person’). The results from both types of assessments are compared with legal limits. The effective doses (defined in Appendix 2) are calculated and compared with the legal dose limit of 1mSv per year for members of the public. All legal radiation dose limits in the UK are based on recommendations made by the ICRP (International Commission on Radiological Protection 2007), which are consistent with BSSD 13 (European Commission 2014). The radiation dose specifically to skin is also assessed in some cases and compared with the legal limit for skin exposure.
The radiation doses resulting from human activities may be compared with the exposure from natural radioactivity. The average individual radiation dose in the UK population (in 2010) from natural radiation was estimated by UKHSA to be approximately 2.3mSv per year (Oatway and others, 2016).
Radiation exposures to some specific groups of workers are included in the assessment of doses from nuclear licensed sites. These are people who may be inadvertently exposed as a result of their work. These, for example, include fishermen, farmers, and sewage workers. It is appropriate to compare their doses to the dose limit for members of the public (Allott 2005). Those people who specifically work with ionising radiation have their radiation doses assessed and recorded as part of their employer’s programme to assess occupational exposure (United Kingdom - Parliament 1999a).
1.2.2 ‘Total dose’ results for 2023
The results of the assessment for each site are summarised in Table 1.2 (see also Figure S and Table S in the Technical Summary). These data are presented in 3 parts. The representative person receiving the highest annual doses from the pathways mainly relating to gaseous discharges and direct radiation are shown in part A and those for liquid discharges in part B. Occasionally, the people receiving the highest doses from all pathways and sources are different from those in parts A and B. Therefore, this case is presented in part C. The major contributions to dose are provided. The use of radionuclide concentrations reported at the limits of detection provide an upper estimate of doses calculated for pathways based on these measurements. The full output from the assessment for each site can be provided by contacting one of the agencies listed on the inside front or back covers of this report.
In all cases, doses estimated for 2023 were much less than the annual limit of 1mSv for members of the public. The people most affected from gaseous discharges and direct radiation varied from site to site but the dominant pathway was often direct radiation (from the relevant site), where it was applicable. The people most affected from liquid discharges were generally adults eating seafood or people who spend long periods of time over contaminated sediments, which are coastal or other areas that are impacted by liquid discharges.
The representative person who received the highest annual ‘total dose’ (0.23mSv), is from the Cumbrian coastal community (near Sellafield), who consumed crustaceans at high rates, together with other seafood. The ‘total dose’ (from all sources) at this site is combined with the effects of all local sources, including specifically the effects of historical discharges of natural radionuclides from the former phosphate processing plant near Whitehaven. The next highest annual ‘total doses’ were received by people living near the Capenhurst (0.15mSv), and Amersham (0.089mSv) sites; these doses were almost entirely due to direct radiation from the sites and were a small fraction of the dose limit.
1.2.3 ‘Total dose’ trends
A time-series of annual ‘total dose’ from 2012 to 2023 is shown in Figure 1.1 (Table 1.3 gives numerical values). Figure 1.1 displays the ‘total dose’ trends at UK nuclear licensed sites over the period 2012 to 2023. More detailed plots are presented in the relevant site texts. In all cases, the assessed ‘total doses’ were less that the 1mSv dose limit to members of the public. More detailed plots are given in the relevant site sections and supporting texts. Many sites showed a downward trend in ‘total dose’ over this period. Changes in direct radiation dominated the variation (from year to year) at most of the power station sites, and small variations in external dose rates had relatively large effects at some sites where intertidal occupancy (time spent on beaches and mud/salt marsh areas) were recorded at high rates. After Magnox reactors (and to a lesser extent AGRs) stopped power generation (for example, at Dungeness), direct radiation has reduced at these sites.
Figure 1.1. ‘Total doses’ around the UK’s nuclear sites due to radioactive waste discharges and direct radiation (from left to right, 2012 to 2023). (Exposures at Sellafield/ Whitehaven/LLWR receive a significant contribution to the dose from technologically enhanced naturally occurring radionuclides from previous non-nuclear industrial operations).
The most significant trend in annual ‘total dose’ due to discharges of waste was for high-rate consumers of seafood in the Cumbrian coastal community (near Sellafield, the former phosphate processing plant near Whitehaven and the LLWR near Drigg). In this case, the overall downward trend in ‘total dose’ broadly followed the general downward trend in concentrations of naturally occurring and artificial radionuclides from non-nuclear and nuclear sources, respectively. Year to year changes in radiation doses were also influenced by changes in consumption and occupancy characteristics of local people and the natural variability in radionuclide concentrations in food and the environment. In recent years, doses to these people have varied due to small differences in the concentrations of polonium-210 in local seafood.
The estimate of the annual ‘total dose’ at Dounreay has decreased in recent years from the peak value in 2008 (Table 1.3). The increase in ‘total dose’ at Dounreay in 2016, 2018, 2021 and 2023 was mostly due to the concentration of caesium-137 found in venison (game) being included, which had not been sampled in previous years. The changes in ‘total dose’ at Heysham (2016), Hinkley Point (2017), and Trawsfynydd (2018) were largely due to findings from new habits surveys. At Springfields, the increase in ‘total dose’ in recent years was due to higher estimates of direct radiation. At Capenhurst, any changes in annual ‘total doses’ over time are attributable to changes in the estimates of direct radiation from the site. The small increases in ‘total dose’ at Bradwell and Winfrith in recent years were mostly due to higher estimates of direct radiation from the individual sites. In all cases doses were well below the legal limit of 1mSv.
1.2.4 Source specific dose results for 2023
The results of the source specific assessments for the main industrial sites in the UK are summarised in Figure 1.2. and Table 1.4, which contains numerical data and the major contributors to dose. Figure 1.2. shows the assessed source specific doses for 2023, both from gaseous and liquid sources, compared to the 1mSv dose limit to members of the public. Doses ranged from less than 0.005mSv (at many sites) to 0.36mSv from liquid sources near Sellafield. These assessments focus on the effect of gaseous or liquid waste discharges, unlike the assessment of ‘total dose’ which includes all sources including the effect of direct radiation.
Figure 1.2. Source specific doses in the UK, 2023. (Exposures at Whitehaven and Sellafield receive a significant contribution to the dose from technologically enhanced naturally occurring radionuclides from previous non-nuclear industrial operations). Part A is from liquid discharges and part B is from gaseous discharges. Small doses less than or equal to 0.005mSv are recorded as being 0.005mSv.
Site name | Dose from liquid discharges, mSv |
---|---|
Sellafield, Whitehaven and LLWR near Drigg | 0.36 |
Berkeley/Oldbury | 0.041 |
Barrow | 0.040 |
Heysham | 0.021 |
Hinkley | 0.020 |
Hartlepool | 0.015 |
Holy Loch | 0.011 |
Winfrith | 0.010 |
Capenhurst | 0.006 |
Springfields | 0.006 |
Trawsfynydd | 0.006 |
Dounreay | 0.006 |
Rosyth | 0.006 |
Aldermaston | 0.005 |
Amersham | 0.005 |
Bradwell | 0.005 |
Derby | 0.005 |
Devonport | 0.005 |
Dungeness | 0.005 |
Harwell | 0.005 |
Sizewell | 0.005 |
Wylfa | 0.005 |
Chapelcross | 0.005 |
Faslane | 0.005 |
Hunterston | 0.005 |
Torness | 0.005 |
limit | 1 |
Site name | Dose from gaseous discharges. mSv |
---|---|
Trawsfynydd | 0.034 |
Dounreay | 0.017 |
Amersham | 0.013 |
Barrow | 0.013 |
Sellafield | 0.012 |
Chapelcross | 0.009 |
LLWR near Drigg | 0.007 |
Heysham | 0.006 |
Hinkley | 0.006 |
Hunterston | 0.006 |
Torness | 0.006 |
Aldermaston | 0.005 |
Berkeley/Oldbury | 0.005 |
Bradwell | 0.005 |
Capenhurst | 0.005 |
Derby | 0.005 |
Devonport | 0.005 |
Dungeness | 0.005 |
Hartlepool | 0.005 |
Harwell | 0.005 |
Sizewell | 0.005 |
Springfields | 0.005 |
Winfrith | 0.005 |
Wylfa | 0.005 |
Faslane | 0.005 |
limit | 1 |
The most significant exposures from seafood (fish and crustacean shellfish) consumption were to the Cumbrian coastal community (at the LLWR near Drigg, and close to Sellafield and the former phosphate processing plant near Whitehaven). The majority of the dose was from non-nuclear industrial operations, resulting in TENORM and, to a much lesser extent, the legacy of historical discharges from Sellafield. The most important pathways and radionuclides at each site were similar to those found for ‘total dose’.
Although some source specific doses were estimated to be higher than ‘total doses’, the reasons for this are understood and relate to the different assumptions of the 2 assessment approaches. The assumptions used for source specific assessments are conservative with respect to adding together the effects of consumption of different foods. The assumptions used for ‘total dose’ assessments are more realistic, and the estimates from the source specific assessments provide reassurance that the ‘total dose’ approach is reasonable. Radiation doses to all age groups (see Section 2.6 for the age groups used), calculated using the source-specific method, were all found to be well below the legal limit of 1mSv per year.
1.2.5 Protecting the environment
This report focusses on the risk to the public (in other words, to ensure that radiation doses remain below limits). However, the environment agencies also consider the protection of wildlife and the environment from radiation exposure caused by human activity. The 2007 recommendations of the ICRP concluded that a systematic approach to the radiological assessment of non-human species was required to support the management of radiation effects in the environment (International Commission on Radiological Protection 2007). The ICRP, therefore, introduced the concept of Reference Animals and Plants (RAPs) for a system of radiological environmental protection (International Commission on Radiological Protection 2008). The ICRP has published its aims covering:
- prevention or reduction of the frequency of deleterious (harmful) radiation effects on biota (animals and plants) to a level where they would have a negligible impact on the maintenance of biological diversity
- the conservation of species and the health and status of natural habitats, communities, and ecosystems (International Commission on Radiological Protection 2014)
In the UK, the current legislative measures for protection of wildlife from radiation are retained from the European Commission directives on the conservation of wild birds (Commission of the European Community 2009) and the conservation of natural habitats and wild flora and fauna (Commission of the European Community 1992). These are implemented through The Conservation of Habitats and Species (Amendment) (EU Exit) Regulations 2019, known as the ‘Habitats Regulations’ (UK Statutory Instruments 2019).
Under the ‘Habitats Regulations’, the Environment Agency, NRW and SEPA are required to review existing authorisations/permits to ensure that no authorised activity or permission has an adverse effect, either directly or indirectly, on the integrity of Natura 2000[footnote 9] habitat sites. Similarly, for any new or varied authorisation/permit, whereby the applicant must assess the potential impact of the discharges on reference organisms that represent species which may be adversely affected.
The Environment Agency has assessed the dose rates to reference organisms and feature species for permitted radioactive waste discharges. It has concluded that the radiation dose to the worst affected organism was less than the agreed dose guideline (40μGy h-1) and therefore, there was likely to be no significant impact on the integrity of habitat sites or their conservation objectives (Environment Agency 2009a; Environment Agency 2009b). The assessment of impacts on wildlife and plants (non-human) species is also an essential part of the Environment Agency’s determination of applications for new and varied environment permits. Further information concerning assessment of dose rates to reference organisms is available in earlier RIFE reports (for example, (Environment Agency and others, 2017)).
SEPA has carried out a pressures and impacts assessment from radioactive substances on Scotland’s water environment. The study concluded that there was no adverse impact on the aquatic environment as a result of authorised discharges of radioactive substances, although it recognised that there may be a need for further data to support this conclusion. SEPA has included a specific habitats assessment in any new authorisation granted by the agency.
SEPA’s nuclear power generation and decommissioning sector plan is available on SEPA’s website: SEPA nuclear power generation and decommissioning sector plan.
1.3 Sources of radiation exposure
1.3.1 Radioactive waste disposal from nuclear licensed sites
The permits[footnote 10] and authorisations issued by the environment agencies to nuclear sites require operators to minimise the amount of all forms of radioactive waste generated. They also limit any liquid or gaseous discharges and ensure that solid low-level waste (LLW) is sent to a suitable disposal site.
Solid LLW from nuclear licensed sites may be transferred to the LLWR near Drigg for a range of treatments or disposal. Solid wastes containing low quantities of radioactivity can also be disposed of at permitted landfill sites (see Section 7). Solid LLW from Dounreay, intended for disposal, can be transferred to the Dounreay LLW facility.
Figure 1.3 shows the nuclear licensed sites, and their respective nuclear sectors, that produce waste containing artificial radionuclides, across the UK. Each site is discussed in the following sections. Nuclear licensed sites are permitted/authorised to dispose of radioactive waste and are also subject to the Nuclear Installations Act 1965 (United Kingdom - Parliament 1965). The monitoring programmes reported here cover all these sites.
In April 2024, Magnox Limited changed its name to Nuclear Restoration Services Limited (NRS) continuing its role as a wholly owned subsidiary of the Nuclear Decommissioning Authority (NDA). For the purposes of the reporting period in this RIFE report, we will maintain reference to Magnox as the operator for the decommissioning legacy power and research sites.
Figure 1.3. Principal nuclear site sources of radioactive waste disposal in the UK, 2023. (Showing main initial operation. Some operations are undergoing decommissioning).
Discharges of radioactive waste from other non-nuclear sites such as hospitals, industrial sites and research establishments were also regulated under RSA 93 or successor legislation (currently EPR 16 in England and Wales, EASR18 in Scotland and still RSA 93 in Northern Ireland), but not subject to the Nuclear Installations Act. Occasionally, radioactivity is detected in the environment during monitoring programmes because of discharges from these other sites. For example, iodine-131 discharged from hospitals is occasionally detected in river and marine samples. Small amounts of very low level solid radioactive waste are disposed of from some non-nuclear sites to approved landfill sites (for controlled burial, incineration, or other treatment/disposal methods). There is also a significant radiological impact due to historical discharges of radionuclides from non-nuclear industrial activity that also occur naturally in the environment. This includes radionuclides discharged from the former phosphate processing plant near Whitehaven, and so monitoring is carried out near this site.
Discharges from other non-nuclear sites are generally considered insignificant in England and Wales, so the environment agencies do not usually carry out monitoring to protect public health. However, some routine monitoring programmes are undertaken in Lancashire and Northamptonshire (Section 7). In Scotland, SEPA carries out routine sampling in the Firth of Clyde and at landfill sites to assess the impact of the non-nuclear industry on the environment. Additionally, to ensure the doses from combined discharges to a sewer network are assessed properly, SEPA periodically undertakes intensive sampling at major sewage treatment plants to monitor the combined discharges from the non-nuclear industry.
Principal permitted/authorised discharges, disposals of radioactive wastes and solid waste transfers from nuclear establishments in 2023, are given in Appendix 1 (Table A1.1 to Table A1.4, inclusive). The tables also list the main discharge and disposal limits that are specified or, in the case of the MOD, administratively agreed. In 2023, discharges and disposals were all below the limits. Solid waste transfers from nuclear establishments in Scotland are also given in Appendix 1 (Table A1.4). Section 7 gives information on discharges from non-nuclear sites.
The discharge limits are set through an assessment process, initiated either by the operator or the relevant environment agency. In support of the process, prospective assessments of doses to the public are made assuming discharges at the specified limits. Using this conservative assumption, discharge limits are set so that doses to the public will be below the source and site dose constraints of 0.3 and 0.5mSv per year, respectively (Environment Agency, Scottish Environment Protection Agency and others, 2012). The determination of discharge limits considers a comprehensive range of pathways including the consumption of food. When determining the limits, the effect of the planned discharges on the environment and wildlife is also taken into account. In addition, the regulations require BAT, under the Environmental Permitting (England and Wales) Regulations, to be used to ensure that discharges and their impact are minimised. The principles of best practicable means (BPM) continue to be applied in Scotland (Scottish Environment Protection Agency 2019b). The UK environment agencies consider that the terms BPM and best practicable environmental option are equivalent to the use of BAT (Scotland & Northern Ireland Forum for Environmental Research 2005; Scottish Environment Protection Agency 2019b).
The discharges and disposals made by sites do not normally fluctuate significantly. However, from time to time there may be unplanned events that cause unintended leakages, spillages or other emissions that are different to the normal or expected pattern of discharges. These events must be reported to the environment agencies and may lead to follow up action, including reactive monitoring by the site, the environment agencies, or the food standards agencies. In cases where there has been a breach of limits, or if appropriate actions have not been carried out to ensure discharges are minimised, regulatory action may be taken. Where monitoring took place because of these events, the results are presented and discussed in the relevant site text later in this report. Appendix 1 (Table A1.5) summarises the types of events that occurred in 2023.
1.3.2 International agreements and new build
This section gives information on the context of UK radioactive discharges as they relate to international agreements and the future building of new nuclear power stations.
International agreements
The UK is a contracting party to the Convention for the Protection of the Marine Environment of the North-East Atlantic (the ‘OSPAR Convention’). This provides a framework for preventing and eliminating pollution in the north-east Atlantic, including the seas around the UK (OSPAR 2000a). In 1998, UK government ministers agreed a long-term Radioactive Substances Strategy (RSS) and signed the Sintra statement which included the following commitment (OSPAR 1998):
“We shall ensure that discharges, emissions and losses of radioactive substances are reduced by the year 2020 to levels where the additional concentrations in the marine environment above historical levels, resulting from such discharges, emissions, losses, are close to zero.”
A UK Strategy for Radioactive Discharges was published in 2002 (Department for Environment Food & Rural Affairs 2002), to describe how the UK would implement the ministerial agreements reached at the 1998 and subsequent meetings of OSPAR. This strategy was revised in 2009 to include gaseous discharges, from decommissioning as well as operational activities, and from the non-nuclear as well as the nuclear industry sectors (Department of Energy and Climate Change and others, 2009). A number of objectives (including the UK’s obligations, with respect to the OSPAR RSS intermediate objective for 2020) and outcomes were identified in the revised strategy. These are summarised in earlier RIFE reports (for example, (Environment Agency, Food Standards Agency, Food Standards Scotland, and others, 2016)).
To support implementation of UK government policy concerning the regulation of radioactive discharges into the environment, the Environment Agency, DESNZ and the Scottish and Welsh Governments (collectively and individually) have issued guidance and developed environmental principles. These are also summarised in earlier RIFE reports (for example, (Environment Agency, Food Standards Agency, Food Standards Scotland, Natural Resources Wales, and others, 2018)).
In 2018, the UK government published its review of the 2009 UK strategy for radioactive discharges (Department for Business Energy and Industrial Strategy 2018). This 2018 review takes account of developments in UK government policy, commercial decisions within the nuclear industry, technological advances, and improvements in our knowledge of the impacts of radionuclides in the marine environment. This review demonstrates the clear evidence of progress being made by the UK in meeting the outcomes of the 2009 strategy and contributing towards the objectives of the OSPAR RSS. Further information and a copy of the report is available on the UK government website: 2018 review of the 2009 discharge strategy.
Information on the approach and work in progress within the OSPAR Convention can be found on OSPAR’s website OSPAR’s website. A recent report from the OSPAR Radioactive Substances Committee records work completed and planned, relating to reporting of discharges, environmental measurements, standards, and quality assurance (OSPAR 2024a). The agreement on monitoring (Coordinated Environmental Monitoring Programme), relevant to OSPAR, was revised (OSPAR 2017). The OSPAR monitoring programme includes sampling in 15 sub-divisions of the OSPAR maritime area as defined in the Second Periodic Evaluation (OSPAR 2007) and is supported by procedures for ensuring quality control. Inputs in the north-east Atlantic have been summarised for both nuclear and non-nuclear sectors (OSPAR 2024b; OSPAR 2024c). The UK submission concerning the implementation of the principle of using BAT has been submitted to OSPAR for publication. The Fifth Periodic Evaluation (OSPAR 2022), represents the final assessment against the objectives of the RSS and formed the basis of the most recent OSPAR Quality Status Report, which was published in September 2023 OSPAR QSR 2023. It demonstrated that Contracting Parties have successfully fulfilled the objectives of the OSPAR RSS for 2020 under the North-East Atlantic Environment Strategy (NEAES) 2010 – 2020 and have made significant progress towards fulfilling the ultimate aim of radionuclide concentrations in the environment near background values for naturally occurring radionuclides and close to zero for artificial radionuclides (OSPAR 2022).
In October 2021, the Contracting Parties to OSPAR, which includes the UK, agreed the NEAES 2030 (OSPAR 2021a) and signed the Cascais Declaration (OSPAR 2021b), setting OSPAR’s strategic direction up to 2030. The NEAES includes the new strategic objective (S3):
“OSPAR will prevent pollution by radioactive substances in order to safeguard human health and to protect the marine environment, with the ultimate aim of achieving and maintaining concentrations in the marine environment at near background values for naturally occurring radioactive substances and close to zero for human made radioactive substances.”
The work for NEAES 2030 will be taken forward through the delivery of 4 operational objectives:
S3.O1: On an ongoing basis, OSPAR will further prevent, progressively reduce or, where that is not practicable, minimise discharges of radioactive substances through the application of BAT, taking into account technical feasibility, radiological impact, and legitimate uses of the sea.
S3.O2: By 2025, OSPAR will identify and consider any obstacles in achieving further reductions in environmental concentrations of radioactive substances in the marine environment and examine possible solutions where appropriate.
S3.O3: By 2025, OSPAR will identify the different types of loss of radioactive substances that may contribute to pollution of the marine environment. By 2027, OSPAR will determine if any additional measures are required to prevent such pollution, to the extent that such pollution is not already the subject of effective measures agreed by other international organisations or prescribed by other international conventions.
S3.O4: By 2028, OSPAR will, following the outcome of the Quality Status report 2023, address, where appropriate, any uncertainties by reviewing and updating methodologies to better determine the possible impact of releases, emissions, and losses of radioactive substances on marine ecosystems.
The importance of an integrated approach to stewardship of the marine environment has long been established in the UK. The reports ‘Safeguarding Our Seas’ (Department of Agriculture Environment and Rural Affairs, Scottish Executive & Welsh Assembly Government 2002) and ‘Charting Progress 2’ (Department for Environment Food & Rural Affairs 2010), provided an initial strategy and assessment on the state of the UK seas. Further information concerning other individual and fully integrated assessments is available in earlier RIFE reports (for example, (Environment Agency, Food Standards Agency, Food Standards Scotland, Natural Resources Wales, and others, 2018)).
In 2010, the Marine Strategy Regulations 2010 came into force. These Regulations require us to take action to achieve or maintain good environmental status (GES) in our seas (subject to certain exceptions) through the production of a “Marine Strategy” for all UK waters and that this is coordinated across the 4 UK Administrations. The UK Marine Strategy provides the framework for assessing and taking measures to achieve and maintain GES in our seas. It covers a wide range of biodiversity and marine environment descriptors including contaminants, and contaminants in seafood.
The UK published an initial assessment of UK seas in 2012 (Part One of the UK Marine Strategy) (Her Majesty’s Government 2012), followed by publication of Part Two, setting out the UK marine monitoring programmes, and Part Three, our Programme of Measures, in 2014 and 2015, respectively (Department for Environment Food & Rural Affairs and others, 2014; Department for Environment Food & Rural Affairs and others, 2015). In October 2019, following a public consultation, the UK published an update to the UK Marine Strategy Part One. It includes an assessment of progress towards the achievement of GES for UK seas and sets out revised targets and indicators for the next 6 years (Department for Environment Food & Rural Affairs and others, 2019). The updated UK Marine Strategy Part Two, which sets out the monitoring programmes that we will use to assess the status of UK seas in respect to these targets and indicators was published in March 2021 (Department for Environment Food & Rural Affairs 2021). The UK Marine Strategy Part Three, which sets out our Programmes of Measures designed to help us achieve or maintain GES, is currently being updated. Further details on the UK Marine Strategy can be found on: marine online assessment tool.
Nuclear new build
In the 2008 white paper ‘Meeting the Energy Challenge’ (Department of Business Enterprise and Regulatory Reform 2008), the UK government set out its view that new nuclear power stations should have a role to play in this country’s future energy mix. More information about the basis of the white paper, subsequent national policy statements, consultations, and decisions, together with details of the approach for assessing potential new nuclear power station designs and approvals for their proposed developments, is available in earlier RIFE reports (for example, (Environment Agency, Food Standards Agency, Natural Resources Wales, and others, 2014)). The UK government has further set out its current position on energy policy in the December 2020 white paper, ‘Powering our Net Zero Future’ (Department of Business Energy and Industrial Strategy (BEIS) 2020). In this white paper, the UK government highlights the need to address climate change urgently and it sets out the strategy for wider energy systems to achieve the UK’s target of net zero greenhouse gas emissions by 2050. The strategy includes a continuing and future role for nuclear generation to provide reliable clean electricity and it sees a potential additional role for advanced modular reactors (AMR) to provide high temperature process heat in the future. In early 2023, DESNZ published a policy paper, describing the various advance nuclear technologies currently under consideration, and available from Advanced Nuclear Technologies on www.gov.uk.
The 8 nuclear sites, assessed as being potentially suitable for the development of new nuclear power stations in the 2011 national policy statement on nuclear power generation (Department of Energy & Climate Change 2011), are shown in Figure 1.4. There are 3 sites on the east coast of the UK, Hartlepool, Sizewell and Bradwell, with 3 more around the Irish Sea, Wylfa, Moorside and Heysham. The last 2 potential sites are in the Bristol Channel at Berkeley and Oldbury. However, only Hinkley Point C and Sizewell C are being actively pursued. The UK government re-affirmed its position on nuclear power generation as part of the 2022 British Energy Security Strategy, with an aim of generating up to 25% of the projected 2050 UK demand through deployment of civil nuclear (HM Government 2022). In March 2023 the government published its ‘Powering Up Britain’ documents that include its Energy Security Plan. This sets out the steps it is taking to ensure that the UK is more energy independent, secure and resilient. It confirms the aim to make final investment decisions on 2 new nuclear power stations in the next parliament (in other words by 2029). It also confirmed that government will develop a new Nuclear National Policy Statement. The documents are available from: Powering up Britain. In January 2024, the UK government published its ‘Civil Nuclear: Roadmap to 2050’ (Department for Energy Security and Net Zero 2024), which sets the governments ambition for up to 24 Gigawatts of nuclear capacity, which would represent 25% of the country’s projected electricity demand. This would be delivered by Great British Nuclear through a combination of large-scale, small and advanced modular reactors.
Figure 1.4. Potential sites for new nuclear power stations.
As regulators of the nuclear industry, the ONR, the Environment Agency and NRW, are working together to ensure that any new nuclear power stations built in the UK meet high standards of safety, security, environmental protection, and waste management through the generic design assessment process (GDA). The steps undertaken by the regulators are described in previous RIFE reports (Environment Agency, Food Standards Agency and others, 2021). More information on the GDA process can be found here: new nuclear power stations assessing reactor designs.
Following a request from DESNZ ministers, the ONR, the Environment Agency and NRW began the GDA process on a 470MWe Small Modular Reactor (SMR) in April 2022. The requesting party for this GDA is Rolls-Royce SMR Ltd. An outline 53-month programme for the three-step assessment process has been proposed for this GDA. The proposed design completed the first step of the GDA in April 2023, with the second step completed in late July 2024. In early October 2023, the UK government selected 6 companies (EDF, GE-Hitachi Nuclear Energy International LLC, Holtec Britain Limited, NuScale Power, Rolls Royce SMR and Westinghouse Electric Company UK Limited) to advance to the next stage of the SMR competition. Following requests from DESNZ ministers, the ONR, the Environment Agency and NRW began the GDA process on 2 further SMR designs, the Holtec International SMR-300 (in October 2023) and the GE Hitachi Nuclear Energy BRWX-300 (in January 2024). Both developers have requested a 2-step GDA process, the Holtec International SMR-300 and the GE Hitachi Nuclear Energy BWRX-300 are at step 2 (August 2024) and step 1, respectively. Further information will be reported in subsequent RIFE reports.
The construction of NNB Generation Company (HPC) Limited new twin UK European Pressurised Reactor™ (EPR™) nuclear power station continues at Hinkley Point C in Somerset. The ONR continues to be engaged in carrying out safety and security assessments and regulating its construction. The Environment Agency also continues to regulate environmental matters at the site under the environmental permits it has granted, including for construction-related discharges. The development of NNB Generation Company (HPC) Limited is of interest to both regulators to ensure that the company has the competences and resources required to secure safety, security, and environment protection throughout construction and as it prepares itself to be an operator.
The ONR and the Environment Agency are continuing to work with Sizewell C Limited (previously NNB GenCo (SZC) Limited) that is seeking to construct a new nuclear power station at Sizewell C, Suffolk based upon UK EPR™ reactor and replication of the Hinkley Point C station.
In 2020, the ONR and Environment Agency received applications from Sizewell C Limited for a nuclear site licence and radioactive substances activities permit. In March 2023, the Environment Agency granted the radioactive substances permit to Sizewell C Limited following public consultation. In May 2024, the nuclear site licence application was granted by the ONR.
1.3.3 Managing radioactive liabilities in the UK
The UK government has been managing radioactive waste for many decades in accordance with the Joint Convention on the Safety of Spent Fuel Management and on the Safety of Radioactive Waste Management (International Atomic Energy Agency 1997). This convention aims to ensure that individuals, society, and the environment are protected from the harmful effects of ionising radiation, from the management of spent nuclear fuel and radioactive waste. Further information relevant to the UK demonstrating compliance under the Joint Convention is available in earlier RIFE reports (for example, (Environment Agency, Food Standards Agency, Food Standards Scotland, Natural Resources Wales and others, 2019)).
The Nuclear Decommissioning Authority (NDA), a non-departmental public body, manages the decommissioning and clean-up of the civil public sector nuclear sites. The NDA reports to DESNZ and is responsible to Scottish ministers. The role of the NDA is strategic, developing and implementing an overall strategy for cleaning up the civil public sector nuclear legacy. The NDA’s strategic objective is to manage radioactive waste and dispose of it where possible, or place it in safe, secure, and suitable storage, in line with the UK and devolved administrations’ policies. The Energy Act 2004 (United Kingdom - Parliament 2004) requires the NDA to review and publish its strategy every 5 years. Its most recent strategy was published in 2021 (Nuclear Decommissioning Authority 2021) and the business plan for 2024 to 2027 is available (Nuclear Decommissioning Agency 2024). In 2019, the NDA published an inventory and forecast of radioactive wastes in the UK (as of 1 April 2019) jointly with DESNZ (Nuclear Decommissioning Authority & Department for Business Energy and Industrial Strategy 2019; Nuclear Decommissioning Agency 2023) and a Mission Progress Report in 2023 (Nuclear Decommissioning Agency 2023).
In 2007, the UK government and devolved administrations issued a UK-wide policy document, setting out principles for the long-term management of solid LLW (Department for Environment Food & Rural Affairs, Department of Trade and Industry & the Devolved Administrations 2007). Following the introduction of the LLW policy, the UK LLW Strategy was published in 2010 (Nuclear Decommissioning Authority 2010). A new UK LLW Strategy was published in 2016 (Department of Energy and Climate Change and others, 2016). Some LLW, mostly from non-nuclear sites, and some very low-level radioactive waste is currently disposed of in landfill by controlled burial (Section 7). There is still a large amount of solid LLW that will require disposal. Some will be sent to the LLWR near Drigg. The LLW from Dounreay can be disposed of at the new Dounreay LLW Facility close to the site.
In 2022, Nuclear Waste Services (NWS) was launched, which brings together the operator of the LLWR, Geological Disposal Facility (GDF) developer Radioactive Waste Management Limited (RWM) and the NDA group’s integrated waste management programmes into a single organisation. NWS has assumed responsibility for the development of a GDF.
In 2023, DESNZ ran a consultation on proposals to update and consolidate the policies of the UK government and devolved administrations on the management of radioactive substances and nuclear decommissioning into a single UK-wide policy framework (Department for Energy Security and Net Zero 2023a; Department for Energy Security and Net Zero 2023b). This has been published in May 2024 (Department for Energy Security & Net Zero and others, 2024). This has replaced the previous national policy on radioactive waste management (United Kingdom - Parliament 1995b).
The NDA is responsible for implementing UK and Welsh Government policies on the long-term management of higher activity radioactive waste (HAW) through geological disposal. Scottish Government policy is that the long-term management of HAW should be in near-surface facilities. Facilities should be located as near to the site where the waste is produced as possible. Guidance to site operators and regulatory position statements on the management of HAW on licensed sites has been issued by the Environment Agency, NRW, SEPA and the ONR (Environment Agency, Office for Nuclear Regulation and others, 2021; Office for Nuclear Regulation and others, 2021).
The UK government’s initial framework was set out in the 2008 ‘Implementing Geological Disposal’ white paper for managing HAW in the long-term through geological disposal and includes the possibility of hosting a GDF at some point in the future (Department for Environment Food & Rural Affairs and others, 2008). An updated framework was outlined in the 2014 white paper (as a replacement in England and Northern Ireland) and sets out the policy for managing HAW in the long-term through geological disposal (Department of Business Energy and Industrial Strategy (BEIS) 2018a). Following completion of the initial actions in the 2014 white paper and subsequent consultation, DESNZ published a policy update in 2018 (Department of Energy and Climate Change 2014). This replaces the 2014 white paper in England and also describes the positions of the devolved administrations: radioactive waste management is a devolved policy issue. Therefore, the Scottish Government, Welsh Government and Northern Ireland Executive each have responsibility for determining disposal policy in their respective areas. Further information on devolved administrations’ policies is available on the GOV.UK website: geological disposal facility gdf for high activity radioactive waste.
No specific GDF sites have been selected or are currently under consideration (Department of Business Energy and Industrial Strategy (BEIS) 2018a). However, following a national geological screening exercise, RWM/NWS have re-started the process to engage with communities across England to host a GDF. Further information on the aspects of GDF is also available on the website: geological disposal facility gdf for high activity radioactive waste.
The Committee on Radioactive Waste Management (CoRWM) continues to provide independent scrutiny of the government’s long-term management, storage, and disposal of radioactive waste. CoRWM has published its annual report for 2022 to 2023 (Committee of Radioactive Waste Management 2023a), proposed work programme for 2023 (Committee on Radioactive Waste Management 2023) and position papers on the UK’s uranium inventory and the development of small modular reactors (SMRs) and advanced modular reactors (AMRs) (Committee of Radioactive Waste Management 2023b; Committee of Radioactive Waste Management 2024).
Guidance on requirements for authorisation for geological and near-surface disposal facilities has been published (Environment Agency, Northern Ireland Environment Agency & Scottish Environment Protection Agency 2009; Environment Agency & Northern Ireland Environment Agency 2009; Environment Agency 2012a). SEPA has issued a policy statement on how it will regulate the disposal of LLW from nuclear licensed sites (Scottish Environment Protection Agency 2012) and published joint guidance with the Environment Agency and NRW on the surrender of nuclear site permits that include how potential onsite disposals of radioactive waste should be considered by site operators (Scottish Environment Protection Agency, Environment Agency & Natural Resources Wales 2018). In May 2019, SEPA issued guidance on the decommissioning of non-nuclear facilities (for example, a single laboratory) from radioactive use (Scottish Environment Protection Agency 2020).
Decommissioning of many nuclear sites in Great Britain is currently underway. Following the environment agencies’ consultation on the draft guidance, ‘Guidance on Requirements for Release of Nuclear Sites from Radioactive Substances Regulation’, referred to as ‘GRR’, the operational feedback from the trial use of the guidance was used to refine the structure and clarity of the guidance. This was published in 2018 (Scottish Environment Protection Agency and others,2018). This guidance describes what operators must do to release sites from radioactive substances regulation and is also available via: decommissioning of nuclear sites and release from regulation.
Naturally occurring radioisotopes are enhanced in some wastes (TENORM) and those wastes are subject to existing regulatory systems that are designed to protect human health and the environment. Further information relevant to the UK NORM Waste Strategy, published in 2014, is available in earlier RIFE reports (for example, (Environment Agency, Food Standards Agency, Food Standards Scotland, Natural Resources Wales and others, 2018)).
1.3.4 Solid radioactive waste disposal at sea
In the past, packaged solid waste of low radioactivity concentrations was disposed of deep in the North Atlantic Ocean, the last disposal of this type was in 1982. The UK government announced at the OSPAR Ministerial meeting in 1998 that it was stopping disposal of this material at sea. At that meeting, Contracting Parties agreed that there would no longer be any exception to prohibiting the dumping of radioactive substances, including waste (OSPAR 1998). The environmental impact of the deep ocean disposals was assessed by detailed mathematical modelling and has been shown to be negligible (Organisation for Economic Co-operation and Development Nuclear Energy Agency 1985). Disposals of small amounts of waste also took place from 1950 to 1963 in a part of the English Channel known as the Hurd Deep. The results of environmental monitoring of this area are presented in Section 8 and confirms that the radiological impact of these disposals was insignificant.
In England, the MMO administers a range of statutory controls that apply to marine works on behalf of the Secretary of State for Environment, Food and Rural Affairs. This includes issuing licences under the Marine and Coastal Access Act 2009 (MCAA) (United Kingdom - Parliament 2009) for the disposal of dredged material at sea. In Northern Ireland, Scotland and Wales, licences for disposal of dredged material at sea are the responsibility of DAERA, the Scottish Government (Marine Scotland) and NRW, respectively.
The protection of the marine environment is considered before a licence is issued. Since dredged materials will contain varying concentrations of radioactivity from natural and artificial sources, assessments are carried out, when appropriate, to provide reassurance that there is no significant risk to the food chain or other risk from the disposal. Guidance on exemption criteria for radioactivity in relation to sea disposal is available (International Atomic Energy Agency 1999). The International Atomic Energy Agency (IAEA) has published a system of assessment that can be applied to dredged spoil disposal (International Atomic Energy Agency 2003; International Atomic Energy Agency 2015) and which has been adapted to reflect operational practices in England and Wales (McCubbin & Vivian 2006). In 2023, no new requests were received to apply for additional licences for the disposal of dredged material (containing radioactivity) at sea.
1.3.5 Other sources of radioactivity
There are several other man-made sources of radioactivity that may affect the food chain and the environment. These could include disposals of material from offshore installations, transport incidents, satellite re-entry, releases from overseas nuclear installations and the operation of nuclear-powered submarines. UKHSA has assessed incidents involving the transport of radioactive materials in the UK (Jones & Harvey 2014). UKHSA have also considered the effects of discharges into the marine environment from the oil and gas industry, with the estimated highest individual dose (per head of population) being less than 0.001mSv per year (Harvey, Smith & Cabianca 2010). Submarine berths in the UK are monitored by the MOD (for example, (Defence Science and Technology Laboratory 2023)). General monitoring of the British Isles is carried out as part of the programmes described in this report, to detect any significant effects from the sources above. No such effects were found in 2023. Low concentrations of radionuclides were detected in the marine environment around the Channel Islands (Section 8), and these may be partly due to discharges from the nuclear fuel reprocessing plant at La Hague in France.
The exploration for, and extraction of, gas from shale rock has been investigated in the UK with support from DESNZ. Further details on fracking: developing shale gas in the UK (updated March 2019) are provided on the GOV.UK website: about shale gas and hydraulic fracturing fracking.
This process, along with others for unconventional sources of gas such as coal bed methane, represents a potential source of exposure to naturally occurring radioactivity for the public and workers. The form of the radioactivity could be gaseous, liquid, or solid. Examples of routes of exposure are inhalation of radon gas emissions, and ingestion of water and food where the process has enhanced concentrations of naturally occurring radioactive material (NORM). The environment agencies, FSA or FSS do not currently monitor radioactivity in the environment and food from the exploration and extraction of shale gas.
In November 2019, the UK government announced “an indefinite suspension” of fracking, after a report by the Oil and Gas Authority, an independent subsidiary of DESNZ, found it was not possible to predict the probability or size of tremors caused by the practice. In late 2019, the Scottish Government finalised its policy position of no support for unconventional oil and gas development in Scotland.
As part of the British Energy Security Strategy [65], DESNZ launched a scientific review of shale gas extraction in April 2022 to advise on the latest scientific evidence and developments in relation to shale gas extraction. In September 2022, the UK government lifted its moratorium on UK shale gas production. This will enable developers to seek planning permission where there is local support.
Further information on the previous involvement by each of the environment agencies to support engagement with industry, and other related issues to shale gas extraction, is available in earlier RIFE reports (for example, (Environment Agency, Food Standards Agency, Food Standards Scotland, Natural Resources Wales and others, 2019)).
The Environmental Protection Act 1990 provides the basis for a regulatory regime for identifying and remediating contaminated land. In the UK, there is a duty to inspect land under Part ll A of the Environmental Protection Act 1990, but there must be reasonable grounds for inspecting land for radioactivity. Reasonable grounds are defined in the statutory guidance. Once it is decided that an area is a special site, it is regulated by the environment agencies in their respective areas.
In England and Wales, regulations were extended in 2007 to cover land contaminated with radioactivity originating from nuclear licensed sites. DESNZ issued revised guidance for radioactive contaminated land to local authorities and the Environment Agency in 2012 (Department of Energy and Climate Change 2012). The Environment Agency has issued a series of briefing notes that provide information on land contaminated with radioactivity in England and Wales (Environment Agency 2012b). In 2018, DESNZ carried out a targeted consultation process on proposed updates to the statutory guidance for radioactive contaminated land on behalf of the UK and Welsh Governments. Updates have subsequently been made to the statutory guidance for England, which was published in 2018 (Department of Business Energy and Industrial Strategy (BEIS) 2018b). Clear dose criteria are set for homogeneous and heterogeneous contamination. Also, the risk (probability or frequency of occurrence) of receiving the dose should be considered for the designation of radioactive contaminated land. To date, no site has been legally designated as ‘radioactive contaminated land’ due to radioactivity in England and Wales.
Equivalent legislation for identifying and remediating contaminated land comprising The Radioactive Contaminated Land Regulations (Northern Ireland) 2006 and subsequent amending legislation, issued in 2007 and 2010, exists as Statutory Instruments in Northern Ireland (Statutory Instruments 2007; Statutory Instruments 2010).
In 2007, the Radioactive Contaminated Land (Scotland) Regulations came into force by amending Part ll A of the Environmental Protection Act 1990. SEPA has powers to inspect land that may be contaminated with radioactivity, to decide if land should be identified as radioactive contaminated land and require remediation if considered necessary. Revised Statutory Guidance was issued to SEPA in 2009. This guidance is broadly similar to that issued to the Environment Agency. In Scotland, clear dose criteria are set for homogeneous and heterogeneous contamination. Also, the risk (probability or frequency of occurrence) of receiving the dose should be considered for the designation of radioactive contaminated land. To date, no site has been designated as ‘contaminated land’ due to radioactivity in Scotland.
The contribution of aerial radioactive discharges from UK installations to concentrations of radionuclides in the marine environment has been studied (Department for Environment Food & Rural Affairs 2004). The main conclusion was that aerial discharges do not make a significant contribution to activity concentrations in the marine environment. On occasion, the effects of aerial discharges may be detected in the aquatic environment, and conversely the effects of aquatic discharges may be detected on land. Where this is found, appropriate comments are made in this report.
All sources of ionising radiation exposure to the UK population are reviewed by UKHSA, the most recent report was published in 2016 (Oatway and others, 2016). The most significant source of exposure was from natural radiation (radon and thoron gases). Figure 1.5 provides a breakdown of the exposure to the UK population by source, 48% from radon exposure, 16% from medical exposures, 13% from terrestrial gamma radiation, 12% cosmic radiation and 11% from the intakes of radionuclides, excluding radon. The side bar shows the contributions from nuclear weapons fallout (0.2%), occupational radiation (0.02%) and radioactive discharges (0.01%). The average individual dose from exposure to all significant sources of ionising radiation was estimated to be about 2.7mSv per year, the same as that reported in the previous review (Watson and others, 2005). The dose from radiation in the environment was about 2.3mSv per year, or about 84% of the dose from all sources of radiation. This was dominated by exposure to naturally occurring sources of radiation although there is significant variation across the UK due to local geology and other factors. Only about 0.2% of the annual dose was from artificial sources; and of this, the majority was from radionuclides released during historical testing of nuclear weapons in the atmosphere (global fallout) from the 1950s and 1960s (hereafter referred to as ‘nuclear weapons testing’), with exposure to radionuclides routinely discharged by industry contributing less than 0.01% to the total dose. The average individual dose from medical sources was about 0.4mSv per year, or about 16% of the dose from all sources of radiation. Occupational exposure contributed significantly less than 1% of the dose. Further information, including the most recent breakdown of the average individual dose to the UK population by source of exposure, is available on the UKHSA website:radiation and you.
Figure 1.5. Average UK population exposure from natural and artificial sources of radioactivity (Oatway and others, 2016)
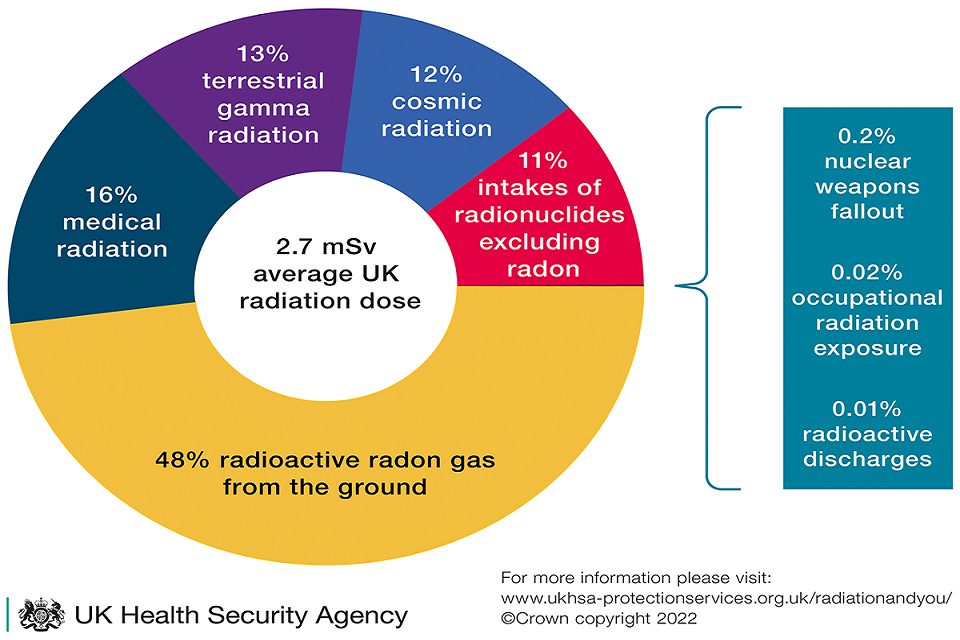
Image courtesy of UK Health Security Agency, @Crown Copyright 2022.
The RIFE report is directed at establishing the exposure of people who might receive the highest possible doses due to regulated radioactive waste discharges, because of their age, diet, location or habits. It is the exposure of these people which forms the basis for comparisons with dose limits in UK law.
2 Methods of sampling, measurement and presentation used in this report
This section explains the scope of the monitoring programmes presented in this report (hereafter referred to as ‘programmes’) and summarises the methods and data used to measure and assess radioactivity in food and the environment. The bulk of the programmes, assessment methods and data have continued in 2023 unchanged, however the main changes are:
Sampling and measurement
- special sampling at nuclear sites – if judged necessary, this was continued where there were unusual short-term increases in discharges and inadvertent releases.
Assessment and presentation
- site maps - maps of sites and sampling locations have been revised and updated
- new habits data - consumption and occupancy rates have been updated with the benefit of recent habits survey results at the LLWR near Drigg and Sellafield in England, Faslane and Torness in Scotland and Wylfa in Wales
Accompanying this report is a further set of files giving full details of each assessment of ‘total dose’ summed over all sources at each site.
The appendices of this report contain further details on:
- modelling to extend or improve the results of monitoring (Appendix 3)
- consumption, occupancy and other habits data (Appendix 4)
- dosimetric data (Appendix 5)
- estimates of concentrations of natural radionuclides (Appendix 6)
Guidance on planning and implementing routine environmental radiological monitoring programmes has been published (Environment Agency and others, 2010; Scottish Environment Protection Agency 2019a). In recent years, the Environment Agency, Food Standards Agency (FSA), Food Standards Scotland (FSS) and Scottish Environment Protection Agency (SEPA) have all completed reviews of their environmental radioactivity monitoring programmes. Further information is available in earlier RIFE reports and in Section 1.1.
2.1 Sampling programmes
The primary purpose of the programmes is to check on the quantities of radioactivity in food and the environment. Results are used to demonstrate that the safety of the public is not compromised and that doses, resulting from discharges of radioactivity, are below the statutory dose limits (see Section 1 for more details). The scope in 2023 extends throughout the UK (and the Channel Islands) and is undertaken independently of the industries which discharge wastes to the environment. Samples of food, water and other materials are collected from the environment and analysed in specialist laboratories. In situ measurements of radiation dose rates and contamination are also made and the results of the programmes are assessed in terms of limits and trends in this report. Subsidiary objectives for the programmes are:
- to provide information to assess the impact on non-human species
- to enable indirect confirmation of compliance with authorised/permitted waste disposals
- to determine whether undisclosed releases of radioactivity have occurred from sites
- to establish a baseline to judge the importance of accidental releases of radioactivity should they occur
- to demonstrate compliance with international obligations, such as The Oslo and Paris Convention for the Protection of the marine environment of the North-East Atlantic (OSPAR)
Routine sampling is focused on nuclear sites licensed under the Nuclear Installations Act, 1965 (United Kingdom - Parliament 1965) since these generally discharge more radioactivity (than from non-nuclear sites) and have a greater influence on the environment. The programmes also serve to provide information to assist the environment agencies to fulfil statutory duties, which are further described in Section 1.1. Additional sampling is conducted in areas remote from nuclear sites to establish the general safety of the food chain, drinking water and the environment. Results from this sampling generate data that are used as ‘background’ activity concentrations to compare with results from around nuclear sites and to show the variation in the quantities of radioactivity across the UK. Quantities of radioactivity in the environment can also be affected by disposals of radioactive waste from nuclear sites abroad and show the legacy of atmospheric fallout from both past nuclear weapons testing and the nuclear reactor accidents (such as at Chernobyl in Ukraine in 1986).
Various methods for undertaking sampling and analysis are available. The programmes are primarily directed at relatively widespread radiological contamination where the likelihood of encounter or consumption is certain. Where a source of potential exposure to particles of radioactivity is concerned, the likelihood of encounter is an important factor. This is considered separately in the main report in site specific programmes targeted at contamination from radioactive particles.
The programmes can be divided into three main sectors, generally based on the source of radioactivity in the environment:
- nuclear licensed sites discharging gaseous and liquid radioactive wastes
- industrial and landfill sites
- UK regional monitoring and overseas accidents
2.1.1 Nuclear licensed sites
Nuclear licensed sites are the prime focus of the programmes as they have been responsible for the largest individual discharges of radioactive waste. Sampling and direct monitoring is conducted close to most of the sites shown in Figure 1.3 (except those which have a very low effect). At Sellafield, radionuclides from liquid discharges can be detected in the marine environment in many parts of north-European waters. Therefore, programmes for this site extend beyond national boundaries.
The frequency and type of measurement and the materials sampled vary from site to site and are chosen to be representative of existing exposure pathways. Information on local peoples’ diets and way of life, from habits surveys, are used to inform the exposure pathways. Consequently, the programme may vary from site to site and from year to year. Detailed information on the scope of the programme at individual sites is given in the tables of results. The routine programme is supplemented by additional monitoring if applicable, for example, in response to incidents or reports of unusual or high discharges of radioactivity with the potential to enter the food chain or the environment. Results of both routine and additional monitoring are included in this report.
The main aim of the programme is to monitor the environment and diet of people who live or work near nuclear sites, to estimate doses for those small groups of people who are most at risk from disposals of radioactive waste. It is assumed that if the most exposed people have a dose below the national and international legal limit then all others should receive an even lower dose. For liquid wastes, the pathways that are the most relevant to discharges are the ingestion of seafood and freshwater fish, drinking water and external exposure from contaminated materials. For gaseous wastes, the effects are due to the ingestion of terrestrial foods, inhalation of airborne activity and external exposure from material in the air and deposited on the ground. Inhalation of airborne activity and external exposure from airborne material and surface deposition are difficult to assess by direct measurement but can be assessed using environmental models. The main part of the monitoring is therefore directed at a variety of foodstuffs and measurements of external dose rates on the shores of seas, rivers and lakes. The programme also includes some important environmental indicators, so that quantities of radioactivity can be put in a historical context.
2.1.2 Industrial and landfill sites
Although the emphasis of the monitoring programme is the nuclear industry, a small proportion of the monitoring programmes are focussed on other activities that may have a radiological impact on people and the food chain. This part of the programme considers the effect of disposals of naturally occurring and artificial radionuclides from non-nuclear industries and of disposal into landfill sites, other than at Dounreay (considered separately in Section 5).
The impact of the non-nuclear industry was studied at several locations in 2023 including East Northants Resource Management Facility (near Kings Cliffe), the River Clyde (Glasgow), Cowpen Bewley (Billingham) and Whitehaven (see Section 7). As in recent years, a small-scale programme was undertaken near Hartlepool (see Section 4.1.1), in addition to that directed at the effects of the power station itself. Sampling and analysis reflected the nature of the sources (of radioactivity) under study and, where appropriate, included consideration of the enhanced concentrations of naturally occurring radionuclides from non-nuclear industrial activity. There are also occasional specific programmes that consider, for example, the effects of land contaminated with historical sources of radioactivity and discharges from non-nuclear sites such as hospitals.
The distribution of landfill sites considered in 2023 is shown in Figure 7.1. Sites were studied to assess the extent of radiological contamination, if any, leaching from the site and re-entering the terrestrial environment (as leachates collected in surface waters close to the sites). The most significant waste disposal site is the Low-Level Waste Repository (LLWR) engineered facility (near Drigg) in Cumbria.
2.1.3 UK regional monitoring and fallout in the UK from overseas accidents
The programme of regional monitoring considers the quantities of radioactivity in the environment in areas away from specific sources as an indication of general radiological contamination of the food supply and the environment. The component parts of this programme are:
- monitoring of the Channel Islands and Northern Ireland
- dietary surveys
- sampling of milk
- drinking water sources, groundwater, rain and airborne particulates
- seawater surveys
- fallout in the UK from overseas accidents
Channel Islands and Northern Ireland
The programmes for the Channel Islands and Northern Ireland are designed to complement that for the rest of the UK and to take into account the possibility of long-range transport of radionuclides.
Channel Islands monitoring is conducted on behalf of the Channel Island States. It consists of sampling and analysis of seafood and indicator materials as a measure of the potential effects of UK and French disposals into the English Channel and historical disposal of solid waste in the Hurd Deep.
Monitoring on the Isle of Man for foodstuffs and indicator materials ceased in 2014 and 2015, respectively. Monitoring of the marine environment is primarily directed at the effects of current and historical disposals from Sellafield.
The Northern Ireland programme is directed at the far-field effects of disposals of liquid radioactive wastes into the Irish Sea. Seafood and indicator materials are collected from a range of coastal locations including marine loughs, and dose rates are monitored on beaches.
General diet
The purpose of the general diet survey programme is to provide information on radionuclides in the food supply to the wider population, rather than to those living near particular sources of radiological contamination such as the nuclear industry. This programme is based on sampling and analysis of canteen meals throughout the UK. It provides background information that is useful in interpreting site-related measurements and helps ensure that all significant sources of radiological contamination form part of the site-related programme. Prior to the UK’s exit from the EU these data were also supplied as part of the UK submission to the EC under Article 36 of the Euratom Treaty[footnote 11] to allow comparison with those from other EU member states (for example, (Commission of the European Community 2000)). While these data are no longer supplied to the EC for England, Wales and Northern Ireland, they will continue to be published in the RIFE reports.
Specific foods, freshwater, rain and airborne particulates
Further background information on the relative concentrations of radionuclides is gained from the sampling and analysis of milk. Freshwater, rain and airborne particulates are also analysed to add to the understanding of radionuclide intakes by the population via ingestion and inhalation and as general indicators of the state of the environment.
Milk sampling took place at dairies throughout the UK in 2022. Prior to the UK’s exit from the EU, milk data were also supplied as part of the UK submission to the EC under Article 36 of the Euratom Treaty to allow comparison with those from other EU Member States. While these data are no longer supplied to the EC for England, Wales and Northern Ireland, they will continue to be published in the RIFE reports.
Meat and crop monitoring of naturally occurring and artificial radionuclides, as a check on general food contamination, remote from nuclear sites, ceased in 2014. However, in 2023, surveillance of imported food at ports of entry using radiation screening equipment continued as a means of detecting the effects of overseas incidents. If screening and subsequent sample analysis shows quantities of radioactivity that fail to comply with UK food standards, which are retained from EU directives/regulations, then the consignments are removed from the UK market.
Freshwater used for the supply of drinking water was sampled throughout England, Northern Ireland, Scotland and Wales. Regular measurements of radioactivity in air and rainwater were also made. Prior to the UK’s exit from the EU, these data were also supplied as part of the UK submission to the EC under Article 36 of the Euratom Treaty to allow comparison with those from other EU Member States. These data will continue to be published in the RIFE reports.
Seawater surveys
Seawater surveys are conducted in the seas around the UK on behalf of the DESNZ to provide information on radionuclide concentrations and information on water transport mechanisms in the coastal seas of northern Europe. Such information is used to support international studies of the health of the seas under the auspices of the OSPAR Conventions (OSPAR 2000b), to which the UK is a signatory and in support of research on the fate of radionuclides discharged to sea. These surveys are conducted using government research vessels and are supplemented by a programme of spot sampling of seawater at coastal locations.
Fallout in the UK from overseas accidents
Monitoring of the long-range effects of the Fukushima Dai-ichi accident started across the UK in March 2011. Samples from all sectors of the environment were taken and analysed by gamma spectrometry. The most significant radionuclides to monitor were iodine-131 and caesium-137, which were prevalent in the release from the accident. Very low activity concentrations were detected, and the extended programme ceased later in 2011. Further details of the programme and the results are given in the RIFE report for 2011 (Environment Agency, Food Standards Agency and others, 2012).
Monitoring of the effects of the 1986 Chernobyl accident was undertaken in relation to the upland contamination of lakes. Earlier RIFE reports have provided detailed results of monitoring by the environment agencies and FSA (Environment Agency and others, 2013). Sheep monitoring ceased in 2012 due to the removal of restrictions on the movement, sale and slaughter of sheep in parts of Cumbria and North Wales. Sampling for freshwater fish in locations affected by Chernobyl ceased in 2014.
2.2 Methods of measurement
There are 2 basic types of measurement made:
- dose rates are measured directly in the environment
- samples collected from the environment are analysed for their radionuclide content in a laboratory
2.2.1 Sample analysis
Analysis of samples varies depending on the nature of the radionuclide under investigation. The types of analysis can be broadly categorised into 2 groups:
- gamma-ray spectrometry
- radiochemical methods
The former is a cost-effective method of detecting a wide range of (gamma-emitting) radionuclides, commonly found in radioactive wastes, and is used for most samples. Radiochemical methods consist of a range of analyses involving the application of chemical separation and purification techniques to quantify the concentrations of alpha- and beta-emitting radionuclides. These are sensitive determinations, but generally more labour intensive. These methods are only used if alpha and beta concentration data are required for specific radionuclides and are not detectable using gamma-ray spectrometry (see Section 2.4 for discussion on limits of detection).
Several laboratories analysed samples in the monitoring programmes described in this report. Their main responsibilities were as follows:
Cefas
Centre for Environment, Fisheries and Aquaculture Science, gamma-ray spectrometry and radiochemical analysis of food samples in England, Wales, Northern Ireland and the Channel Islands.
SOCOTEC
SOCOTEC UK Limited, gamma-ray spectrometry and radiochemical analysis of environmental samples (including analysis of sources of drinking water) in England and Wales.
UKHSA
UK Health Security Agency, gamma-ray spectrometry and radiochemical analysis of food and environmental samples from Scotland, air and rain samples in England, Wales and Northern Ireland, and freshwater for Northern Ireland.
Each laboratory operates quality control procedures to the standards required by the UK environment and food standards agencies and have their analytical procedures independently assessed by the UK Accreditation Service (UKAS), that ensures the requirements from the international standard ISO 17025 (International Organisation for Standardisation 2017) are maintained. Regular calibration of detectors is undertaken and intercomparison exercises are held with participating laboratories. The quality assurance procedures and data are made available to the UK environment agencies, FSA and FSS for auditing. The methods of measurement include alpha and gamma-ray spectrometry; beta and Cerenkov scintillation counting; and alpha and beta counting using proportional detectors.
Corrections are made for the radioactive decay of short-lived radionuclides between the time of sample collection and measurement in the laboratory. This is particularly important for sulphur-35 and iodine-131. If a sample is bulked from a sequence of samples over time, the date of collection of the bulked sample is assumed to be in the middle of the bulking period. Otherwise, the actual collection date for the sample is used. In a few cases where short-lived radionuclides are part of a radioactive decay chain, the additional activity (‘in-growth‘ and equilibrium status) produced from radioactive decay of parent and daughter radionuclides after sample collection is also considered. Where necessary, corrections to the activity present at the time of measurement are made to take account for 2 radionuclides, protactinium-233 and thorium-234.
The analysis of foodstuffs is conducted on that part of the sampled material that is normally eaten, for example, shells of shellfish and the pods of some legumes are discharged before analysis. Most other foodstuffs are analysed raw, as it is conceivable that all the activity could be consumed in the raw foodstuff.
2.2.2 Measurement of dose rates and contamination
Measurements of gamma dose in air over intertidal and other areas are normally made at 1m above the ground using RadEye SX Survey Meters or Mini Instruments[footnote 12] environmental radiation meters type 6-80, with both type of meters connected to compensated Geiger-Muller tubes, type MC-71. For certain important activities, for example, for people living on houseboats or for wildfowlers lying on the ground, measurements at other distances from the ground may be made. External beta doses are measured on contact with the source, for example fishing nets, using Mini Instruments[footnote 12], Smart ION and Electra PB19RD monitors. These portable instruments are calibrated against recognised reference standards and the inherent instrument background is subtracted. There are 2 quantities that can be presented as measures of external gamma dose rate, total gamma dose rate or terrestrial gamma dose rate. Total gamma dose rate includes all sources external to the measuring instrument. Terrestrial gamma dose rate excludes cosmic sources of radiation but includes all others. In this report, the total gamma dose rate is presented. UKHSA reports terrestrial gamma dose rates to SEPA. Terrestrial gamma dose rate is converted to total gamma dose rate by the addition of 0.037µGy h-1 which approximates the contribution made by cosmic radiation (Her Majesty’s Inspectorate of Pollution 1995).
Beta/gamma monitoring of radiological contamination on beaches or riverbanks is undertaken using similar instrumentation to that for measurements of dose rates. The aim is to cover a large area including strandlines where radioactive debris may become deposited. Any item found with activity rates in excess of the action levels is removed for analysis. An action level of 100 counts per second (equivalent to 0.01mSv h-1) is used in England and Wales. At Dalgety Bay and Dounreay, in Scotland, and at Sellafield, in Cumbria, special monitoring procedures are in place due to the potential presence of radioactive particles on beaches. Further information regarding Dalgety Bay and Dounreay, and Sellafield is provided elsewhere in this report.
2.3 Presentation of results
The tables of monitoring results contain summarised values of observations obtained during the year under review. The data are generally rounded to 2 significant figures. Values near to the limits of detection will not have the precision implied by using 2 significant figures. Observations at a given location, for activity concentrations and dose rates, may vary throughout the year. This variability may be due to changes in rates of discharge, different environmental conditions and uncertainties arising from the methods of sampling and analysis.
The method of presentation of the summarised results allows the data to be interpreted in terms of public radiation exposures for comparison with agreed safety standards.
For milk samples, the most appropriate quantity for use in assessments is the arithmetic mean in the year sampled from the farm with the highest mean activity concentration. This is labelled ’max’ in the tables of results to distinguish it from the values that are averaged over a range of farms. For other terrestrial foods, an alternative approach is adopted since it is recognised that the possible storage of foods harvested during a particular time of the year has to be taken into account. Greater public exposures would be observed coincidental with foods being harvested at times of elevated radiological contamination. For such foods, as well as the mean value, the maximum activity concentration (labelled ’max’ in the tables) observed for each radionuclide is presented at any time in the relevant year and forms the basis for the assessment of dose.
Results are presented, where a sample is taken or a measurement is made, for each location or source of supply. Sample collectors are instructed to obtain samples from the same location during the year. Spatial averaging is therefore not generally undertaken, though it is inherent in the nature of some collected samples. A fish may move some tens of kilometres in an environment of changing concentrations in seawater, sediments and lower trophic levels. The resulting quantity of radiological contamination therefore represents an average over a large area. Similarly, cows providing milk at a farm may feed on grass and other fodder collected over a distance of a few kilometres of the farm. In the case of dose rate measurements, the position where the measurement is conducted is within a few metres of other measurements made within a year. Each observation consists of the mean of many instrument readings at a given location.
The numbers of farms that were sampled to provide information on activities in milk at nuclear sites are indicated in the tables of results. Milk samples collected weekly or monthly are generally bulked to provide 4 quarterly samples for analysis each year. Otherwise, the number of sampling observations in the tables of concentrations refers to the number of samples that were prepared for analysis during the year. In the case of small animals such as molluscs, one sample may include several hundred individual animals.
The number of sampling observations does not necessarily indicate the number of individual analyses conducted for a specific radionuclide. In particular, determinations by radiochemical methods are sometimes conducted less frequently than those by gamma-ray spectrometry. However, results are often based on bulking of samples such that the resulting determination remains representative.
2.4 Detection limits
There are 2 main types of results presented in the tables:
- positively detected values
- values preceded by a ‘less than’ symbol (<)
Where the results are an average of more than one value, and each value is positive, the result is positive.
Alternatively, where there is a mixture of data, or all data is at the limit of detection (LoD) or minimum reporting level (MRL), the result is preceded by a ’less than‘ symbol. Gamma-ray spectrometry can provide many ’less than‘ results.
Limits of detection are governed by various factors relating to the measurement method used and these are described in earlier reports (Ministry of Agriculture Fisheries and Food 1995). There are also a few results quoted as ‘not detected’ (ND) by the methods used. This refers to the analysts’ judgement that there is insufficient evidence to determine whether the radionuclide is present or absent.
2.5 Additional information
The main aim of this report is to present all the results of routine monitoring from the programmes described previously. However, it is necessary to carry out some averaging for clarity and to exclude some basic data that may be of use only to those with specific research interests. Full details of the additional data are available from the environment agencies and FSA. Provisional results of concentrations of radionuclides in food samples collected in the vicinity of nuclear sites in England, Northern Ireland (milk and canteen meals) and Wales are available upon request from FSA.
The main categories of additional data are:
- data for individual samples prior to averaging
- uncertainties in measurements
- data for very short-lived radionuclides supported by longer-lived parents
- data which are not relevant to a site’s discharges for naturally occurring radionuclides and for artificial radionuclides below detection limits
- measurements conducted as part of the research programme described in Appendix 7
Very short-lived radionuclides such as yttrium-90, rhodium-103m, rhodium-106m, barium-137m and protactinium-234m (formed by decay of, strontium-90, ruthenium-103, ruthenium-106, caesium-137 and thorium-234, respectively) are taken into account for calculating exposures to members of the public. They are not listed in the tables of results. As a first approximation, their concentrations can be taken to be the same as those of their respective parents.
2.6 Radiation protection standards
The monitoring results in this report are interpreted in terms of radiation exposures of the public, commonly termed ‘doses’. This section describes the dose standards that apply in ensuring protection of the public.
UK practice relevant to the public was based on the recommendations of the ICRP as set out in ICRP Publication 60 (International Commission on Radiological Protection 1991). The dose standards were embodied in national policy on radioactive waste (United Kingdom - Parliament 1995b) and in guidance from the IAEA in their Basic Safety Standards (BSS) for Radiation Protection (International Atomic Energy Agency 1996). Legislative dose standards were contained in the BSS Directive 96/29/Euratom (Commission of the European Community 1996) and were subsequently incorporated into UK law in the Ionising Radiations Regulations 1999 (United Kingdom - Parliament 1999b). To implement the BSS Directive, ministers provided the Environment Agency and SEPA with directions concerning radiation doses to members of the public and their methods of estimation and regulation for all pathways (Department of the Environment Transport and the Regions 2000; Scottish Executive 2000). In Northern Ireland, regulations were made to implement the requirements of the BSS Directive in the Radioactive Substances (Basic Safety Standards) Regulations (Northern Ireland) 2003 (Northern Ireland Assembly 2003). The methods and data used in this report are consistent with these (and subsequent) Directions.
The ICRP issued revised recommendations for a system of radiological protection in 2007 as set out in ICRP Publication 103 (International Commission on Radiological Protection 2007). UKHSA have provided advice on the application of the ICRP 2007 recommendations to the UK (Health Protection Agency 2009). Overall, they consider that the new recommendations do not imply any major changes to the system of protection applied in the UK. In particular, for authorised/permitted releases, limits for effective and skin doses remain unchanged. Dose coefficients are also unchanged until such a time as new values are available and receive legislative endorsement.
ICRP (2007) (International Commission on Radiological Protection 2007) use the term ‘representative person‘ for assessing doses to members of the public. It is defined as ’an individual receiving a dose that is representative of the more highly exposed individuals in the population‘. The new term is equivalent to ’critical group‘ which has been used in some previous RIFE reports. Where appropriate, the term ’representative person‘ has been adopted in this report. The EU has updated the BSS Directive to account for the changes in ICRP recommendations (European Commission 2014). The revised directive, 2013/59/Euratom, was published in 2013 and arrangements for transposition of the Directive into UK law are complete. In 2017, the HSE consulted on the changes to the Ionising Radiations Regulations 1999, aiming to transpose the requirements of the revised Euratom Basic Safety Standards Directive 2013 (BSSD 13) (Directive 2013/59/Euratom). The new Ionising Radiations Regulations 2017 (IRR 17) came into force on 1 January 2018 (United Kingdom - Parliament 1999a), replacing the Ionising Radiations Regulations 1999.
Revised standards in England and Wales concerning radiation doses to members of the public, and their methods of estimation and regulation for all pathways, came into force in 2018 in the Environmental Permitting (England and Wales) (Amendment) (No. 2) Regulations 2018 (EPR 18) (United Kingdom - Parliament 2018). Also, in 2018, the Radioactive Substances (Modification of Enactments) Regulations (Northern Ireland) 2018 and the Environmental Authorisations (Scotland) Regulations 2018 (EASR18) came into force for radioactive substances activities in Northern Ireland (Statutory Rules of Northern Ireland 2018) and in Scotland (Scottish Government 2018). Further changes in UK radiological protection law and standards will be taken into account for future issues of this RIFE report.
The relevant dose limits, for authorised/permitted releases, to members of the public are 1mSv (millisievert) per year for whole-body (more formally ‘committed effective’) dose and 50mSv per year specifically for skin. The latter limit exists to ensure that specific effects on skin due to external exposure are prevented and is applicable, for example, in the case of handling of fishing gear. The dose limits are for use in assessing the impact of direct radiations and controlled releases (authorised/permitted discharges) from radioactive sources. In situations that present a novel exposure pathway for members of the public, ‘potential’ exposure routes and standards are determined, and these are discussed further in relation to particles of radioactivity (Dale, Robertson & Toner 2008). For contamination, known to be due to radioactive particles in the UK, a site-specific assessment is considered in the relevant section of this report.
The mean annual dose received by the ‘representative person’ is compared with the dose limit. The term ‘representative person‘ refers to those people most exposed to radiation. In this report, they are usually members of the public consuming large quantities of locally harvested food (high-rate consumers) or spending long periods of time in locations being assessed for external exposure. The limits apply to all age groups. Children may receive higher doses than adults because of their physiology, anatomy and dietary habits. The embryo/foetus can also receive higher doses than its mother. Consequently, doses have been assessed for different age groups; for example, adults, children (10-year-old), infants (1-year-old) and prenatal children, and from this information it is possible to determine which of these age groups receives the highest doses.
For drinking water, the Water Supply (Water Quality) Regulations 2016 (Statutory Instruments 2016), prescribe the requirements on the quality of water intended for human consumption in respect of radioactive substances and is retained from the 2013 EU Directive (European Commission 2013). These regulations specify values for radon, tritium and ‘Indicative Dose’ (ID) above which UK regulatory bodies shall assess whether the presence of radioactive substances in drinking water poses a risk to human health that requires action and, where necessary, shall take remedial action to improve the quality of water to a level which complies with the requirement for the protection of human health from a radiation protection point of view. The values are concentrations of 100Bq l-1 for radon or tritium and a dose of 0.1mSv from an intake over one year. ID is the sum of the doses from individual radionuclides in drinking water excluding tritium, potassium-40 and radon, and its short-lived decay products. Drinking water is taken to include bottled waters (spring and drinking).
The Water Supply (Water Quality) Regulations (Statutory Instruments 2016) also specifies screening values for gross alpha and beta activity of 0.1 and 1.0Bq l-1, respectively. If concentrations are below these values, further investigations are not needed unless it is known that specific radionuclides are present in the drinking water that are liable to cause an ID in excess of 0.1mSv from an intake over one year. Transposition of the Drinking Water Directive into law has now taken place for the whole of the UK. The Water Supply (Water Quality) Regulations 2016 came into force in 2016 in England and Wales (Statutory Instruments, 2016). Similar regulations have been implemented in, Scotland (Scottish Statutory Instruments 2015), Wales (Welsh Statutory Instruments 2018) and Northern Ireland (Statutory Rules of Northern Ireland 2017).
Accidental releases may be judged against UK and ICRP standards in emergency situations (Commission of the European Community 1987; International Commission on Radiological Protection 2007). In addition, it is government policy that food intervention levels retained from EU standards will be taken into account for setting discharge limits. Guidelines for radionuclides in foods following accidental radiological contamination for use in international trade has been published by the Codex Alimentarius Commission (Committee on Interagency Research and Policy Coordination Alimentarius Commission 2011).
The focus of this report, and radiological regulation and monitoring more generally, is towards protection of humans. However, ICRP in its 2007 recommendations has concluded that there was a need for a systematic approach for the radiological assessment of non-human species to support the management of radiation effects in the environment (International Commission on Radiological Protection 2007). More recently ICRP considered the use of a set of reference animals and plants (RAPs) for dose assessments (International Commission on Radiological Protection 2008) and have now published their aims in terms of environmental protection, that is:
- prevention or reduction of the frequency of deleterious radiation effects on biota to a level where they would have a negligible impact on the maintenance of biological diversity
- the conservation of species and the health and status of natural habitats, communities and ecosystems (International Commission on Radiological Protection 2014). No dose limits are proposed to apply but a set of derived consideration reference levels of dose for representative species are recommended for use in assessing the impact of different sources of exposure.
The Habitats Directive (Commission of the European Community 1992) requires a three-stage approach to the assessment of the impact of radioactive discharges on sensitive habitats. Details are provided in Section 1 of this report.
2.7 Assessment methods
Calculations of exposures to members of the public in this report are primarily based on the environmental monitoring data for the year shown under study. The methods used have been assessed for conformity with the principles endorsed by the UK National Dose Assessment Working Group (Allott 2005), and were found to be compatible (Camplin & Jenkinson 2007). There are 2 types of dose assessment made. The first type gives an estimate of the ‘total dose’ to people around the nuclear sites. It considers the effects of all sources, that is the discharges of gaseous and liquid wastes and direct radiation from sources on the site premises (Camplin and others, 2005). The stages of the method are given below.
- Using the consumption and occupancy data from habits surveys
- Use the ‘cut-off’ method to group individuals whose consumption/occupancy is within a factor of 3 of the observed maximum
- Habits profiles for the group determined by averaging the habits rates of all individuals in the group
- Repeat steps 2 and 3 for all pathways identified in habits survey
- Doses to each profiled group calculated (using concentrations of radionuclides in food and environmental dose rates). The group with the highest dose near each site becomes the representative person
The second type of assessment is focused on specific sources and their associated pathways (see Section 1.2 and Appendix 2 in the main report for additional information). It serves as a check on the robustness of the ‘total dose’ assessment and is also compatible with the approach used prior to the introduction of ‘total dose’ in 2004.
‘Total dose’ assessments include direct radiation. The estimates of direct radiation dose are provided by The Office for Nuclear Regulation (ONR) based on information supplied by industry (Coleby 2024). Both types of assessment provide information on 2 other main pathways:
- ingestion of foodstuffs
- external exposure from contaminated materials in the aquatic environment
Collective doses are beyond the scope of this report. They are derived using modelling techniques. The EC has published an assessment of individual and collective doses from reported discharges from nuclear power stations and reprocessing sites for gaseous and liquid waste disposals from 2004 to 2008 (Jones and others, 2013).
Monitoring data is also used to assess doses from pathways, which are generally of lesser importance:
- drinking water
- inadvertent ingestion of water and sediments
- inhalation of re-suspended soil and sediment
In addition, models are used to supplement the monitoring data in 3 situations:
- atmospheric dispersion models are used for non-food pathways where monitoring is not an effective method of establishing concentrations or dose rates in the environment
- food chain models provide additional data to fill gaps and to adjust for high limits of detection
- modelling of exposures of sewage workers is undertaken for discharges from Aldermaston and Amersham
Full details are given in Appendix 3.
For pathways involving intakes of radionuclides, the data required for assessment are:
- concentrations in foodstuffs, drinking water sources, sediments or air
- the amounts eaten, drunk or inhaled
- the dose coefficients that relate an intake or activity to a dose
For external radiation pathways, the data required are:
- the dose rate from the source, for example a beach or fishermen’s nets
- the time spent near the source
In both cases, the assessment estimates exposures from these pathways for people who are likely to be most exposed.
2.8 Concentrations of radionuclides in foodstuffs, drinking water sources, sediments and air
In nearly all cases, the concentrations of radionuclides are determined by monitoring and are given in the main text of this report. The concentrations chosen for the assessment are intended to be representative of the intakes of the most exposed consumers in the population. All of the positively determined concentrations tabulated are included irrespective of the origin of the radionuclide. In some cases, this means that the calculated exposures could include contributions due to disposals from other sites as well as from fallout from nuclear weapon testing and activity deposited following a nuclear reactor accident (such as at Chernobyl in 1986). Where possible, corrections for ‘background’ concentrations of naturally occurring radionuclides are made in the calculations of dose (see Section 2.12).
For aquatic foodstuffs, drinking water sources, sediments and air, the assessment is based on the mean concentration near the site in question. For milk, the mean concentration at a nearby farm with the highest individual result is used in the dose assessment. This procedure accounts for the possibility that any farm close to a site can act as the sole source of supply of milk to high-rate consumers.
For other foodstuffs, the maximum activity concentrations are selected for the assessment. This allows for the possibility of storage of food harvested at a particular time when the peak quantities in a year may have been present in the environment.
The tables of activity concentrations include ‘less than’ values as well as positive determinations. This is particularly evident for gamma-ray spectrometry of terrestrial foodstuffs. Where a result is presented as a ’less than‘ value, the dose assessment methodology treats it as if it were a positive determination as follows:
- when that radionuclide is specified in the relevant permit/authorisation (gaseous or liquid)
- when that radionuclide was determined using radiochemical methods
- when a positive result is reported for that radionuclide in another sample from the same sector of the environment at the site (aquatic or terrestrial)
Although this approach may produce an overestimation of dose, particularly at sites where activity concentrations are low, it ensures that estimated exposures are unlikely to be understated.
2.9 Consumption, drinking and inhalation rates
2.9.1 Source specific assessments
In the assessment of the effects of disposals of liquid effluents, the amounts of fish and shellfish consumed are determined by site-specific dietary habits surveys. Data are collected primarily by direct interviews with potential high-rate consumers who are often found in fishing communities. Children are rarely found to eat large quantities of seafood, and their resulting doses are invariably less than those of adults. The calculations presented in this report are therefore representative of adult seafood consumers or their unborn children if the prenatal children age group is more restrictive.
In assessments of terrestrial foodstuffs, the amounts of food consumed are derived from national surveys of diet and are defined for 3 age groups: adults, children (10-year-old) and infants (1-year-old) (based on (Byrom and others, 1995)). Adult consumption rates are used in the assessment of doses to prenatal children. For each food type, consumption rates at the 97.5th percentile of consumers have been taken to represent the people consuming a particular foodstuff at a high rate (the consumption rate of the ‘representative person‘).
Drinking and inhalation rates are general values for the population, adjusted according to the times spent in the locations being studied.
The consumption, drinking and inhalation rates are given in Appendix 4. Estimates of dose are based on the most up to date information available at the time of writing the report. Where appropriate, the data from site-specific surveys are averaged over a period of 5 years following the recommendation of the report of the consultative exercise on dose assessments (CEDA) (Food Standards Agency 2001).
The assessment of terrestrial foodstuffs is based on 2 assumptions:
- that the foodstuffs eaten by the most exposed individuals are those that are sampled for the purposes of monitoring
- that the consumption of such foodstuffs is sustained wholly by local sources
The 2 food groups resulting in the highest dose are taken to be consumed at high consumption rates, while the remainder are consumed at mean rates. The choice of 2 food groups at the higher consumption rates is based on statistical analysis of national diet surveys. This shows that only a very small percentage of the population were critical rate consumers in more than 2 food groups (Ministry of Agriculture Fisheries and Food 1996). Locally grown cereals are not considered in the assessment of exposures as it is considered highly unlikely that a significant proportion of cereals will be made into locally consumed (as opposed to nationally consumed) foodstuffs, notably bread.
2.9.2 ‘Total dose’ assessments
The ‘total dose’ assessments are based on consumption and occupancy data collected from site specific surveys which are targeted at those most likely to be exposed around the site. The habits profiles that give rise to the highest doses in the assessment of RIFE data are given in files that accompany this report. Care should be taken in using these data in other circumstances because the profile leading to the highest doses may change if the measured or forecast concentrations and dose rates change.
2.10 Dose coefficients
Dose calculations for intakes of radionuclides by ingestion and inhalation are based on the compendium of dose coefficients taken from ICRP Publication 119 (International Commission on Radiological Protection 2012) and from ICRP 88 (International Commission on Radiological Protection 2001) and National Radiological Protection Board (NRPB) (National Radiological Protection Board 2005). In mid-2024, ICRP launched the consultation on the publication of revised dose coefficients to members of the public (part 2). It is expected that revised dose coefficients to members of the public will be made available over the next few years.
These coefficients (often referred to as “dose per unit intake”) relate the committed dose received to the amount of radioactivity ingested or inhaled. The dose coefficients used in this report are provided in Appendix 5 for ease of reference.
Calculations are performed for 4 age groups: adults, children (10-year-old), infants (1-year-old) and prenatal children as appropriate to the pathways being considered. The prenatal age group was introduced following the publication of recommendations by NRPB in 2005 (National Radiological Protection Board 2005). In RIFE, the dose assessment of the embryo and foetus is from a pregnant ‘representative person’. This assumption is considered reasonable in the context of making comparisons with dose limits because it is difficult to demonstrate otherwise. When applied in practice, the doses estimated for the prenatal group are rarely larger than the values for other age groups.
The dose assessments include the use of appropriate gut uptake factors (proportion of radioactivity being absorbed from the digestive tract). Where there is a choice of gut uptake factors for a radionuclide, we have generally chosen the one that gives the highest predicted exposure. In particular, where results for total tritium are available, we have assumed that the tritium content is wholly in an organic form. However, we have also taken into account specific research work of relevance to the foods considered in this report. This affects the assessments for tritium, polonium, plutonium, and americium radionuclides as discussed in Appendix 5.
2.11 External exposure
In the assessment of external exposure, there are 2 factors to consider:
- the dose rate from the source
- the time spent near the source
In the case of external exposure to penetrating gamma radiation, uniform whole-body exposure has been assumed. The radiation as measured is in terms of the primary quantity known as ‘air kerma rate’, a measure of the energy released when the radiation passes through air. This has been converted into exposure using the factor 1 milligray = 0.85mSv (International Commission on Radiological Protection 2010). This factor applies to a rotational geometry with photon energies ranging from 50keV to 2MeV. This is appropriate for the instrument used whose sensitivity is much reduced below 50keV, and to the geometry of deposits of artificial radionuclides. Applying an isotropic geometry gives a value of 0.70Sv Gy-1 , which would be more appropriate for natural background radiation. The choice of 0.85 will therefore tend to overestimate dose rates for the situations considered in this report which include both artificial and natural radiation.
For external exposure of skin, the measured quantity is radiological contamination in Bq cm-2 . In this case, dose rate factors in Sv y-1 per Bq cm-2 are used, which are calculated for a depth in tissue of 7mg cm-2 (Kocher & Eckerman 1987). The time spent near sources of external exposure are determined by site-specific habits surveys in a similar manner to consumption rates of seafood. The occupancy and time spent handling fishing gear are given in Appendix 4.
2.12 Subtraction of ‘background’ activity concentrations
For assessing internal exposures in seafood due to the ingestion of carbon-14 and radionuclides in the uranium and thorium decay series, ‘background’ activity concentrations are subtracted. Background carbon-14 concentrations in terrestrial foods are also subtracted. The estimates of background activity concentrations are given in Appendix 6. For assessing the artificial effect on external exposures to gamma radiation, dose rates due to background are subtracted. Since measurements made previously as part of the monitoring programmes reported here, the gamma dose rate backgrounds in the aquatic environment are taken to be 0.05µGy h-1 for sandy substrates, 0.07µGy h-1 for mud and salt marsh and 0.06µGy h-1 for other substrates. These data are compatible with those presented in (McKay and others, 1995). However, where it is difficult to distinguish the result of a dose rate measurement from natural background, the method of calculating exposures based on the concentrations of artificial radionuclides in sediments is used (Hunt 1984). Estimates of external exposures to beta radiation include a component due to naturally occurring (and un-enhanced) sources because of the difficulty in distinguishing between naturally occurring and artificial contributions. Such estimates are therefore conservative, compared with the relevant dose limit that excludes natural sources of radiation.
2.13 Uncertainties in dose assessment
Various methods are used to reduce the uncertainties in the process of the dose estimation of the representative person. These address the following main areas of concern:
- programme design
- sampling and in situ measurement
- laboratory analysis
- description of pathways to humans
- radiation dosimetry
- calculational and presentational error
Quantitative estimation of uncertainties in doses is beyond the scope of this report.
3 Nuclear fuel production and reprocessing
Highlights
- ‘total doses’ for the representative person were 23% (or less) of the annual dose limit for all assessed sites. ‘Total doses’ decreased to the Cumbrian coastal community near Sellafield, compared to the values in 2022, but remained well below the legal limit
Capenhurst, Cheshire
- ‘total dose’ for the representative person was 0.15mSv and increased in 2023
Springfields, Lancashire
- ‘total dose’ for the representative person was 0.040mSv and increased in 2023
Sellafield, Cumbria
- ‘total doses’ for the representative person were 0.23mSv (or less) and decreased in 2023
- the highest ‘total doses’ were from seafood, dominated by the effects of naturally occurring radionuclides. Historical discharges from the Sellafield site made a lesser contribution
- radiation dose from historical discharges of naturally occurring radionuclides (non-nuclear industry) was lower in 2023. The contribution to ‘total dose’ from Sellafield discharges increased in 2023
- gaseous discharges of caesium-137, plutonium-alpha and americium-241/curium-242 were slightly higher, in 2023 when compared to those in 2022
- liquid discharges were generally lower, in 2023 when compared to those in 2022
This section considers the results of monitoring, by the Environment Agency, FSA, NIEA and SEPA, of 3 sites in the UK associated with civil nuclear fuel production and reprocessing. These sites are at:
Capenhurst, a site where uranium enrichment is carried out, and management of uranic materials and decommissioning activities are undertaken; Springfields, a site where fuel for nuclear power stations is fabricated; and Sellafield, a site where irradiated fuel was reprocessed from nuclear power stations (this activity ceased during 2022) and a range of decommissioning and legacy waste management activities are being carried out.
The Capenhurst site is owned partly by Urenco UK Limited (UUK) and partly by the NDA. UUK holds the nuclear site licence, and their main commercial business is production of enriched uranium for nuclear power stations. The NDA’s legacy storage and decommissioning activities are managed by Urenco Nuclear Stewardship Limited (UNS), a company of the Urenco Group. Another Urenco Group company, Urenco ChemPlants Limited (UCP), are the operators of the Tails Management Facility. This new plant, on a separate part of the site, opened in 2019 and is currently undergoing commissioning.
Both the Springfields and Sellafield sites are owned by the NDA. The Springfields site is leased long-term to Springfields Fuels Limited (SFL) and used to carry out nuclear fuel manufacture and other commercial activities. SFL have a contract with the NDA to decommission legacy facilities on the site and process legacy NDA materials. The main operations on the Sellafield site are decommissioning and clean-up of redundant nuclear facilities, and waste treatment and storage. In 2016, the NDA became the owner of Sellafield Limited, the site licensed company responsible for managing and operating Sellafield on behalf of the NDA, replacing the previous management model of ownership (parent body organisation (PBO) concept) by the private sector
Windscale was historically a separate licensed site located on the Sellafield site, with 3 nuclear reactors. In 2017, Windscale was amalgamated into the Sellafield nuclear licensed site and Sellafield environmental permits.
Decommissioning activities are continuing at Windscale. Most of the radioactive wastes derive from decontamination and decommissioning operations. Gaseous wastes are permitted from specific stacks from Windscale, and liquid radioactive wastes are discharged to the Irish Sea via the Sellafield site pipelines. Both gaseous and liquid discharges are included as part of the permitted Sellafield discharges. Discharges of both gaseous and liquid radioactive wastes are minor compared to those from other Sellafield discharges. Regular monitoring of the environment by the Environment Agency and FSA in relation to any legacy releases from Windscale is conducted as part of the overall programme for the Sellafield site.
Gaseous and liquid discharges from each of these sites (Capenhurst, Springfields and Sellafield) are regulated by the Environment Agency. In 2023, gaseous and liquid discharges were below permit limits for each of the sites (see Appendix 1, Table A1.1 and Table A1.2). Data tables and results of radiological dose assessments for these sites, in Open Document Spreadsheet (ODS) format are downloadable from the main RIFE page.
3.1 Capenhurst, Cheshire
The Capenhurst site is located near Ellesmere Port and is home to uranium enrichment plants and associated facilities. The major operators at the site are UUK, UNS and UCP. UUK operates 3 plants producing enriched uranium for nuclear power stations. UNS manages assets owned by the NDA, comprising uranic material storage facilities and activities associated with decommissioning, UNS also manage radioactive wastes on behalf of all 3 operators. UCP are currently commissioning a new facility, to allow safer long-term storage of depleted uranium, on a separate part of the site. This facility, the Tails Management Facility, de-converts uranium hexafluoride (UF6 or “Hex”) to uranium oxide (U3O8). This allows the uranium to be stored in a more chemically stable oxide form for potential future reuse in the nuclear fuel cycle. This process recovers hydrofluoric acid for reuse in the chemical industry. The plant is permitted for radioactive substances activities and discharges gaseous waste to the environment, aqueous waste to UUK’s effluent disposal system and will dispose of solid waste by off-site transfer.
The operators on the Capenhurst site each have their own environmental permits. The UCP permit was varied in 2020 and the UNS permit was varied in 2020. These variations amended routes for disposal by transfer of liquid wastes for UCP tails facility and revised the discharge limits for UNS.
The most recent habits survey to determine the consumption and occupancy rates by members of the public was undertaken in 2021 (Moore, Clyne & Greenhill 2022).
Doses to the public
The ‘total dose’ from all pathways and sources of radiation was 0.15mSv in 2023 (Table 3.1), or 15% of the dose limit to members of the public. This is an increase from 0.14mSv in 2022. The increase in ‘total dose’ was mainly attributed to a higher estimate of direct radiation from the site, in comparison to that in 2022. This dose was almost entirely due to direct radiation from the Capenhurst site. The representative person was an adult living close to the site, a change from 2022 (a child exposed to direct radiation close to the site). The trend in annual ‘total dose’ over the period 2012 to 2023 is given in Figure 3.1. ‘Total doses’ at Capenhurst ranged from 0.095mSV in 2012 to 0.15mSv in 2023 and all were below the dose limit to members of the public. Any changes in annual ‘total doses’ over time were due to changes in the estimates of direct radiation from the site.
Figure 3.1. ‘Total dose’, in mSv y-1, at Capenhurst, 2012 to 2023.
Year | Dose, mSv y-1 |
---|---|
Dose limit | 1.0 |
2012 | 0.085 |
2013 | 0.080 |
2014 | 0.17 |
2015 | 0.13 |
2016 | 0.17 |
2017 | 0.17 |
2018 | 0.16 |
2019 | 0.17 |
2020 | 0.17 |
2021 | 0.17 |
2022 | 0.14 |
2023 | 0.15 |
Source specific assessments for high-rate consumers of locally grown foods, and for children playing in and around Rivacre Brook, give exposures that were less than the ‘total dose’ in 2023 (Table 3.1). The dose for children (10-year-old), who play in and around the brook and may inadvertently ingest water and sediment, was 0.006mSv in 2023 (up from 0.005mSv in 2022). The increase in dose was due to higher gamma dose rates measured over the riverbank at Rivacre Brook in 2023. The dose is estimated using cautious assumptions for occupancy of the bank of the brook, inadvertent ingestion rates of water and sediment, and gamma dose rates.
Gaseous discharges and terrestrial monitoring
Uranium is the main radioactive constituent of gaseous discharges from Capenhurst, with small amounts of other radionuclides present in discharges by UNS. The focus for terrestrial sampling was the analyses of technetium-99 and uranium in food (including milk), grass and soil. Results for 2023 are given in Table 3.2(a). Concentrations of radionuclides in milk and food samples around the site were very low and generally similar to those in previous years. As in 2022, isotopes of uranium in silage were enhanced by small amounts (most likely due to natural variation) in 2023. Figure 3.2 shows the trends over time (2012 to 2023) of low technetium-99 discharges and corresponding technetium-99 concentrations in grass. The overall trend reflects the reductions in discharges of technetium-99 from the enrichment of reprocessed uranium in the past. The most recently observed variability (from year to year) in the technetium-99 concentrations is based on data reported as less than values. Discharges of uranium are also given in Figure 3.2 and are low over the period 2012 to 2023.
Figure 3.2. Discharges of gaseous and liquid radioactive wastes and monitoring of the environment, Capenhurst (2012 to 2023). (Note different scales used for discharges and activity concentrations).
Liquid waste discharges and aquatic monitoring
The permit for the UUK Capenhurst site allows liquid waste discharges to the Rivacre Brook for uranium and uranium daughters, technetium-99 and non-uranium alpha (mainly neptunium-237). Monitoring included the collection of samples of fish and shellfish from the local aquatic and downstream marine environment (for analysis of a range of radionuclides) and of freshwater and sediments for the analysis of tritium, technetium-99, gamma-emitting radionuclides, uranium, neptunium-237, and gross alpha and beta. Dose rate measurements were taken on the banks of the Rivacre Brook and surrounding area. Results for 2023 are given in Table 3.2(a) and Table 3.2(b). Concentrations of radionuclides in foods from the marine environment were very low and generally similar to those in previous years. The concentrations in fish and shellfish reflect the distant effects of discharges from Sellafield.
As in previous years, sediment samples collected downstream from the Rivacre Brook contained very low but measurable concentrations of uranium (enhanced above natural concentrations) and technetium-99. As expected, enhanced concentrations of these radionuclides (and others) were measured close to the discharge point (Rivacre Brook). Technetium-99 and uranium radionuclide concentrations from this location were lower in 2023, in comparison to those in 2022, but similar to those in other recent years. Variations in concentrations in sediment from the brook are also to be expected due to differences in the size distribution of the sedimentary particles. Concentrations of radionuclides in freshwaters at the discharge point (and at other freshwater locations) were very low in 2023. Measured gamma dose rates near to the discharge point were higher in 2023, in comparison to those in 2022 (where comparisons can be made for similar ground types and locations). Downstream of the Rivacre Brook, at the location where children play, dose rates over grass were also higher in 2023.
Figure 3.2 also shows the trends over time of the releases of several other permitted radionuclides and activity concentrations in environmental samples. These include uranium, ‘uranium daughters’ and ‘non-uranic alpha’ discharges and during the period 2012 to 2023, the overall trend was a reduction of liquid discharges over time. Most of the reductions attributed to progress in decommissioning of some older plant and equipment. During the period 2012 to 2023, the overall trend was a reduction of liquid discharges over time, with most of the reductions attributed to progress in decommissioning of some older plant and equipment.
Concentrations of technetium-99 in sediment (Rivacre Brook) from liquid discharges were detectable close to the discharge point in 2023. The trend of technetium-99 in sediment is given in Figure 3.2 and were enhanced due to the proximity of the discharge outfall (Rivacre Brook), concentrations in 2017 were higher than adjoining years as discussed in RIFE 23 (Environment Agency, Food Standards Agency, Food Standards Scotland, Natural Resources Wales and others, 2018). Concentrations of caesium-137 and americium-241 in sediments at Rock Ferry on the Irish Sea coast were from past discharges from Sellafield carried into the area by tides and currents. The concentrations were generally similar over most of the time period and any fluctuations were most likely due to the effects of normal dispersion in the environment. The lowest activity concentrations at Rock Ferry were reported in 2016.
3.2 Springfields, Lancashire
The Springfields site at Salwick, near Preston, is operated by SFL under the management of Westinghouse Electric Company UK Limited, on behalf of the NDA. The main commercial activity is the manufacture of fuel elements for nuclear reactors and the deconversion of uranium hexafluoride to produce powders and pellets for other fuel manufacturers. Other important activities include recovery of uranium from residues and decommissioning redundant plants and buildings, under contract to the NDA, who retain responsibility for the historical nuclear liabilities on the site.
Research and development, carried out by the National Nuclear Laboratory, produces small amounts of other gaseous radionuclides that are also discharged under permit (see Appendix 1, Table A1.1).
Monitoring around the site is carried out to check not only for uranium concentrations, but also for other radionuclides discharged in the past (such as actinide decay products from past discharges when uranium ore concentrate (UOC) was the main feed material) and for radionuclides discharged from Sellafield. The monitoring locations (excluding farms) used to determine the effects of gaseous and liquid discharges are shown in Figure 3.3. The corresponding sample types and locations are listed in the corresponding site tables.
Figure 3.3. Monitoring locations at Springfields, 2023 (not including farms).
The most recent habits survey was undertaken in 2022 (Clyne and others, 2023). In the 2022 survey, no houseboat dwellers were recorded, therefore this assessment was discontinued. Figures for consumption rates, together with occupancy and handling rates, are provided in Appendix 4 (Table A4.2).
Doses to the public
The ‘total dose’ from all pathways and sources of radiation was 0.040mSv in 2023 (Table 3.1), or approximately 4% of the dose limit to members of the public. This is an increase from 0.032mSv in 2022. The increase in ‘total dose’ was mainly attributed to a higher estimate of direct radiation from the site, in comparison to that in 2022. In 2023, the representative person was an adult living near to the site and unchanged from 2022. Most of the dose to the representative person was from direct radiation. The trends of ‘total dose’ over the period 2012 to 2023 are given in Figure 3.4 and are generally low over this period, the increase observed in 2019 was due to a higher estimate of direct radiation caused by increased occupancy of some workers undertaking a specific responsibility (associated with the rail line) close to the site.
Source specific assessments give exposures that were all less than the ‘total dose’ in 2023 (Table 3.1) for:
- consumers of locally grown food and of seafood (including wildfowl)
- farmers spending time on the banks of the estuary
- children playing on the banks of the estuary
No houseboat occupancy was observed during the 2022 habits survey. Trends in source-specific doses for a high-rate houseboat dweller are presented in earlier RIFE reports.
Figure 3.4. ‘Total dose’, in mSv y-1, at Springfields (2012 to 2023).
Year | Dose, mSv y-1 |
---|---|
Dose limit | 1.0 |
2012 | 0.068 |
2013 | 0.060 |
2014 | 0.050 |
2015 | 0.050 |
2016 | 0.038 |
2017 | 0.028 |
2018 | 0.075 |
2019 | 0.14 |
2020 | 0.047 |
2021 | 0.031 |
2022 | 0.032 |
2023 | 0.040 |
The dose for high-rate consumers of seafood and wildfowl was 0.006mSv in 2023, with approximately 0.005mSv from external exposure (the remainder being from consumption of fish, crustaceans and wildfowl) and slightly lower than that in 2022 (0.007mSv). The most important radionuclides were caesium-137 and americium-241 from past discharges from the Sellafield site.
A source specific assessment for external exposure to farmers was less than 0.005mSv in 2023 (Table 3.1) and unchanged from 2022. The estimated doses to high-rate consumers of locally grown food, and to children playing on the banks of the estuary were all less than 0.005mSv in 2023.
It has been previously shown that assessed annual doses to the public from inhaling sediment from the Ribble Estuary, re-suspended into the air, were much less than 0.001mSv, and negligible in comparison with other exposure routes (Rollo and others, 1994).
Gaseous discharges and terrestrial monitoring
Uranium is the main radioactive constituent of gaseous discharges, with small amounts of other radionuclides present in discharges from the National Nuclear Laboratory’s (NNL) research and development facilities.
The focus of the terrestrial sampling was for the analyses of tritium, carbon-14, strontium-90, iodine-129, and isotopes of uranium, thorium, plutonium and americium in milk and vegetables. Grass, soil and freshwater samples were collected and analysed for isotopes of uranium. Data for 2023 are given in Table 3.3(a). Uranium isotope concentrations in beetroot were similar to those in 2022. Concentrations of thorium were also low in vegetable and grass samples. As in previous years, elevated concentrations of uranium isotopes were measured in soils around the site, but the isotopic ratio showed that they were most likely to be naturally occurring. Overall, results were broadly similar to those of previous years.
Figure 3.5 shows the trends over time (2012 to 2023) of gaseous uranium discharges and total uranium radionuclide concentrations in food (cabbage; 2012 to 2013: beetroot; 2014 to 2023). Over the period, uranium discharges have declined, with the lowest value reported from this site in 2022. Total uranium was detected in cabbage and beetroot samples during the period, as given in Figure 3.5, but the concentrations were very low. The apparent peak of uranium in beetroot in 2017 was also low and significantly less than that found in soil samples.
Figure 3.5. Discharges of gaseous and liquid radioactive wastes and monitoring of the environment, Springfields 2012 to 2023. (Note different scales used for discharges and activity concentrations).
Liquid waste discharges and aquatic monitoring
Permitted discharges of liquid waste (including gross alpha and beta, technetium-99, thorium-230, thorium-232, neptunium-237, uranium and ‘other transuranic radionuclides’) are made from the Springfields site to the Ribble Estuary via 2 pipelines. All discharges were slightly lower in 2023, in comparison to those in 2022. Compared to previous years, discharges are now generally lower for beta-emitting radionuclides. This includes the short half-life beta-emitting radionuclides (mostly thorium-234) that have decreased following the end of the UOC purification process in 2006. Process improvements in the uranium hexafluoride production plants on the Springfields site have reduced the amounts of other uranium compounds needing recycling. These improvements, alongside a reduction in legacy uranic residue processing, have led to a corresponding reduction in discharges of uranium in recent years. Discharges of technetium-99 depend almost entirely on which legacy uranic residues are being processed. Since completion of one particular residue processing campaign (in 2012), technetium-99 discharges have generally declined, with the lowest value (reported as <1% of the annual limit) from this site in 2021. The Ribble Estuary monitoring programme consisted of ‘in situ’ dose rate measurements, the collection and analysis of sediments for uranium and thorium isotopes and gamma-emitting radionuclides.
Results for 2023 are shown in Table 3.3(a). As in previous years, radionuclides due to discharges from both Springfields and Sellafield were detected in sediment and biota in the Ribble Estuary. Radionuclides found in the Ribble Estuary originating from Sellafield were technetium-99, caesium-137 and americium-241. Isotopes of uranium and the short half-life radionuclide thorium-234 were also found from Springfields. Concentrations of the latter were closely linked to recent discharges from the Springfields site. In 2023, thorium-234 concentrations in sediments (over the range of sampling sites) were generally similar compared to those in 2022. Over a much longer timescale these concentrations have declined due to reductions in discharges as shown by the trend of sediment concentrations at the outfall, Lower Penwortham and Becconsall (Figure 2.5, (Environment Agency, Food Standards Agency, Food Standards Scotland, Natural Resources Wales and others, 2018)). The most significant change in the discharge trends was the step reduction of short half-life beta-emitting radionuclides in liquid discharges, mostly thorium-234. The reduction was because the UOC purification process ended in 2006. In more recent years, thorium-234 concentrations have generally declined by small amounts in sediments at Lower Penwortham and Becconsall (Figure 3.5), with the lowest values reported at Lower Penwortham and the outfall in 2023.
Caesium-137 and americium-241 radionuclides were found in biota and sediments from the Ribble Estuary in 2023. The presence of these radionuclides was due to past liquid discharges from Sellafield, carried from west Cumbria into the Ribble Estuary by sea currents and adsorbed on fine-grained muds. The concentrations observed were generally similar to those in recent years.
Figure 3.5 also provides trend information over time (2012 to 2023) for a number of other permitted radionuclides and activity concentrations in food, flounder and shrimp. Liquid discharges of uranium radionuclides steadily decreased (and other discharges to a lesser extent) over the whole period, whilst technetium-99 discharges generally decreased overall (but peaked in 2012 and 2017). Caesium-137 concentrations in flounder showed variations between years and this was most likely due to natural changes in the environment, although there is evidence of decreasing concentrations overall. Technetium-99 concentrations in shrimp showed variations between years and this was most likely due to natural changes in the environment, with lower concentrations observed as discharges have decreased in recent years.
Gamma dose rates (Table 3.3(b)) in the estuary were generally higher than expected natural background rates (see Section 2.12), and this is due to Sellafield-derived gamma-emitting radionuclides (caesium-137 and americium-241). In 2023, gamma dose rates in the estuary, were generally higher (by small amounts) to those in 2022, but with some small variations at some sites. Beta dose rates over salt marsh (where comparisons can be made) were similar to those in recent years.
3.3 Sellafield, Cumbria
Sellafield Limited is responsible for the operation of the Sellafield site and is a wholly owned subsidiary of the NDA. In 2023, the main operations on the Sellafield site were:
- post-operational clean out at the Magnox reprocessing facility
- the decommissioning and clean-up of redundant nuclear facilities
- waste treatment and storage.
The site also contains the Calder Hall nuclear power station, Magnox and the Thermal Oxide Reprocessing Plant (THORP), which are all undergoing decommissioning.
Nuclear fuel reprocessing at THORP ceased in 2018, resulting in reduced gaseous and liquid discharges in the intervening period. THORP will continue to serve the UK until the 2070’s as a storage facility for spent AGR fuel. In July 2022, the Magnox reprocessing facility took its final feed of spent nuclear fuel marking the end of 58 years of Magnox reprocessing. The facility will now enter the post-operational clean-out phase. The Sellafield site also contains the Calder Hall Magnox nuclear power station, which ceased generating in 2003 and is undergoing decommissioning.
The environmental permit for receipt and disposal of radioactive waste was revised and re-issued to the site operator in October 2023 (Environment Agency 2023). Further details of the revised permit can be found in previous RIFE reports (for example, (Environment Agency, Food Standards Agency and others, 2021)). The changes to the environmental permit include:
- registration of the Magnox Swarf Storage Silo (MSSS) retrievals ventilation system (RVS)
- registration of an Outfall X to discharge construction related aqueous waste arisings (non-sewage trade waste) to the River Calder via surface water drainage.
- the removal of the Gaseous Annual Site Limits for Krypton-85 & Antimony-125 following the completion of Magnox reprocessing in July 2022.
- a reduction to the Radium-226 limit and update to the site plan due to Sellafield Ltd.’s application to extend the Calder Landfill Extension Segregated Area (CLESA) into an adjacent ‘valley area’. Please note that there are no required changes to the site’s Radioactive Substances Activities (RSA) environmental permit site boundary.
With the completion of Magnox reprocessing in July 2022 (and a period of post-operational clean out), the gaseous and liquid discharge limits relating to this waste stream have reverted to the lower discharge limits as described in the permit documentation (Environment Agency 2020; Environment Agency 2021). The remaining gaseous limits are upper limits, which are in force until the active commissioning of HEPA filtration in the MSSS stack as detailed in the footnotes of Table A1.1.
The gaseous discharge limits presented in Table A1.1 for strontium-90, caesium-137, plutonium-alpha and americium-241 and curium-242 are the upper limits, which are in force until the active commissioning of High-Efficiency Particulate Air (HEPA) filtration in the MSSS stack as detailed in the footnotes of Table A1.1. All of the lower liquid limits are in force after completion of Magnox reprocessing (Table A1.2).
Sellafield Limited continued retrievals of sludge from legacy pond facilities in 2023 and continues to prepare for retrievals of intermediate level waste from legacy facilities to reduce environmental risk. Some of these projects have the potential to impact on discharges to the environment.
A full habits survey is conducted every 5 years in the vicinity of the Sellafield site, which investigates the exposure pathways relating to liquid and gaseous discharges, and to direct radiation. Annual review surveys are also undertaken between these full habits surveys. These annual surveys investigate the pathways relating to liquid discharges, review high-rate fish and shellfish consumption by local people (part of the Cumbrian Coastal Community group) and review their intertidal occupancy rates. The most recent full Sellafield habits survey was conducted in 2023 (Moore and others, 2024a). A full survey around the LLWR near Drigg was also performed in 2023 (Moore and others, 2024b). In 2023, some changes were found in the amounts (and mixes) of seafood species consumed and intertidal occupancy rates (Moore, Clyne & Greenhill 2023). Revised figures for consumption rates, together with occupancy rates, are provided in Appendix 4 (Table A4.2). Further afield, the most recent habits surveys were conducted to determine the consumption and occupancy rates by members of the public on the Dumfries and Galloway coast in 2017 (Smith and others, 2021) and around Barrow and the south-west Cumbrian coast in 2012 (Garrod CJ and others, 2013a). The results of these surveys are used to determine the potential exposure pathways, related to liquid discharges from Sellafield in Cumbria.
Habits surveys to obtain data on activities undertaken on beaches relating to potential public exposure to radioactive particles in the vicinity of the Sellafield nuclear licensed site were undertaken in 2007 and 2009 (Clyne and others, 2008; Clyne and others, 2010).
An important source of naturally occurring radionuclides in the marine environment has been the phosphate processing plant near Whitehaven in Cumbria. Although the plant closed in 1992, the effects of these past operations continue due to the decay products of the long-lived parent radionuclides discharges to sea. Naturally occurring radionuclides from this (non-nuclear) industrial activity are also monitored and assessed (see Section 7.5). The discharge effects from the Sellafield site and the former phosphate works both influence the same area and therefore the contributions to doses from both sources are considered in Section 3.3.1.
Monitoring of the environment and food around Sellafield reflects the historical and present-day Sellafield site activities. In view of the importance of this monitoring and the assessment of public radiation exposures, the components of the programme are considered here in depth. The discussion is provided in 4 sub-sections, relating to the assessment of dose, the effects of gaseous discharges, the effects of liquid discharges and unusual pathways of exposure identified around the site.
3.3.1 Doses to the public
‘Total dose’ from all pathways and sources
The annual ‘total dose’ from all pathways and sources of radiation is assessed using consumption and occupancy data from the full habits survey of 2023 (Moore and others, 2024c) and the yearly review of shellfish and fish consumption, and intertidal occupancy in 2022 (Moore and others, 2023). Calculations are performed for 4 age groups (adults, 10-year-old children, 1-year-old infants and prenatal children). The effects on high-rate consumers of fish and shellfish from historical discharges of naturally occurring radionuclides from non-nuclear industrial activity from the former phosphate works near Whitehaven (see Section 7.5) are included to determine their contribution to the annual ‘total dose’. These works were demolished in 2004 and the authorisation to discharge radioactive wastes was revoked. The increase in concentrations of naturally occurring radionuclides (due to TENORM) from historical discharges is difficult to determine above a variable background (see Appendix 6).
In 2023, the highest ‘total dose’ to the Cumbrian coastal community[footnote 13], near Sellafield, was assessed to have been 0.23mSv (Table 3.16), or 23% of the dose limit to members of the public. This is a decrease from 0.24mSv in 2022. This dose includes a contribution from sources of direct radiation on the LLWR site near Drigg (included as a result of the 2023 LLWR habits survey (Moore and others, 2024b)). As in previous years, most of this dose was due to radioactivity from sources other than those resulting from Sellafield discharges, in other words, from historical discharges of naturally occurring radionuclides from past non-nuclear industrial activity. The representative person was an adult consuming crustacean shellfish at high rates who also consumed significant quantities of other seafood (fish) and unchanged from 2022. The decrease in ‘total dose’ in 2023 was mostly attributed to the revision of habits information particularly the reduced consumption rate of both fish and crustacean species. Polonium-210 (and lead-210) are important radionuclides as small changes in concentrations above the natural background of these radionuclides, significantly influence the dose contribution from these radionuclides (due to a relatively high dose coefficient used to convert an intake of radioactivity into a radiation dose) and therefore the value of the estimated dose.
Direct radiation from the Sellafield and LLWR near Drigg sites (0.004mSv and 0.033mSv, Table 1.1) in 2023 was considered in the ‘total dose’ assessments, but this made a minor contribution to the highest ‘total dose’ (at LLWR near Drigg).
The most significant contributors to the ‘total dose’ in 2023 were from crustacean consumption (93%), direct radiation (from the LLWR site) (5%), fish consumption (2%) and other pathways (1%). The listed food groups are consumption pathways, the other pathways include external exposure over sediment and saltmarsh. The most important radionuclide was polonium-210 (91%) with transuranic radionuclides (including plutonium-239+240 and americium-241) contributing less than 2% of the dose.
The dose in 2023 from artificial radionuclides discharged by Sellafield (including external radiation) and from historical discharges of naturally occurring radionuclides (from past non-nuclear industrial activity) contributed 0.019mSv and 0.21mSv, respectively[footnote 14]. In 2022, the contributions were 0.014mSv and 0.22mSv, respectively. In 2023, the contribution from external radiation was 0.001mSv (0.002mSv in 2022). Data for naturally occurring radionuclides in fish and shellfish, and their variation in recent years, are discussed in Section 7.5.
The contribution to the ‘total dose’ of 0.019mSv in 2023 from artificial radionuclides (including external radiation) was higher, in comparison to that in 2022. The increase in the contribution to the ‘total dose’ from 2023 was mostly attributed to the contribution from sources of direct radiation from the LLWR near Drigg site (approximately 0.010mSv) The contributing radionuclides in 2023 were mostly iodine-129 (15%), americium-241 (15%) and plutonium radionuclides (3%). External exposure contributed 61% of the ‘total dose’ from artificial radionuclides (20% in 2022).
The contribution to the ‘total dose’ of 0.21mSv in 2023 from naturally occurring radionuclides (from past non-nuclear industrial activity) was lower in comparison to that in 2022 (0.22mSv). In 2023, the most contributing radionuclide was polonium-210 (~99%). The decrease in ‘total dose’ in 2023 was mostly attributed to the revision of habits information particularly the reduced consumption rate of both fish and crustacean species. In 2022, polonium-210 concentrations (above expected background) in locally caught lobsters contributed 0.19mSv to the ‘total dose’. Polonium-210 concentrations (above expected natural background) in fish samples contributed 0.001mSv to the ‘total dose’ in 2023.
Contributions to the highest annual ‘total dose’ each year (2012 to 2023), from all pathways and sources by specific radionuclides, are given in Figure 3.6. Inter-annual variations were more complex and governed by both natural variability in seafood concentrations and real changes in the consumption and occupancy characteristics of the local population. Over a longer period, the trend is of generally declining dose (Figure 2.6, (Environment Agency, Food Standards Agency, Food Standards Scotland, Natural Resources Wales, and others, 2018)).
Figure 3.6. Contributions to ‘total dose’ from all sources at Sellafield, 2012 to 2023. (The highest ‘total dose’ in 2013 due to Sellafield discharges was to people living on houseboats near Barrow in Cumbria).
The largest proportion of the ‘total dose’, from 2014 to 2020, was due to enhanced naturally occurring radionuclides (from past non-nuclear industrial activity) and a smaller contribution from the historical discharges from Sellafield. In 2013, the highest ‘total dose’ (relating to the effects of Sellafield) was entirely due to external radiation from sediments. The change was due to both decreases in polonium-210 (a naturally occurring radionuclide from past non-nuclear industrial activity at the former phosphate processing plant near Whitehaven) and a revision of habits information, resulting in a change in the representative person. In the following year (2014), the increase in ‘total dose’ was due to a change in the habits information from the most recent survey. Thereafter, the relative changes in dose were largely due to variations in polonium-210 concentrations in locally caught lobsters and crabs.
The contributions, from all pathways and sources, to the highest annual ‘total dose’ from the non-nuclear and nuclear industries, and, for adults only, from each pathway of exposure, are also given in Figure 3.7 (2012 to 2023) and Figure 3.8 (2019 to 2023), respectively. The overall trend from the nuclear industry is a generally declining dose (Figure 3.7), broadly reflecting a general reduction in concentrations in seafood of artificial radionuclides from the nuclear industry, over the period 2012 to 2023. The pathways of exposure contributing the highest dose were mollusc, crustacean and sea fish consumers.
Figure 3.7. Contributions from nuclear and non-nuclear industries to ‘total dose’ from all sources at Sellafield, 2012 to 2023. (The highest ‘total dose’ in 2013 due to Sellafield discharges was to people living on houseboats near Barrow in Cumbria).
Figure 3.8. Contributions from each pathway of exposure to the ‘total dose’ from all sources, 2019 to 2023.
Other age groups received less exposure than the adults ‘total dose’ of 0.23mSv in 2023 (10-year-old children: 0.13mSv; 1-year-old infants: 0.088mSv; prenatal children: 0.037mSv). ‘Total doses’ estimated for each age group may be compared with the dose for each person of approximately 2.7mSv to members of the UK population from exposure to natural radiation in the environment (Oatway and others, 2016) and to the annual dose limit to members of the public of 1mSv.
‘Total dose’ from all pathways and sources in the vicinity of the Sellafield site
The highest total dose for a local high-rate seafood consumer was assessed to have been 0.22 mSv in 2023,or approximately 22 per cent of the dose limit to members of the public (Table 3.16). The representative person was an adult consuming crustacean shellfish at high rates who also consumed significant quantities of other seafood (fish) and unchanged from 2022. The decrease in ‘total dose’ in 2023 was mostly attributed to the revision of habits information particularly the reduced consumption rate of both fish and crustacean species. Polonium-210 (and lead-210) are important radionuclides as small changes in concentrations above the natural background of these radionuclides, significantly influence the dose contribution from these radionuclides (due to a relatively high dose coefficient used to convert an intake of radioactivity into a radiation dose) and therefore the value of the estimated dose.
Direct radiation from the Sellafield (0.004mSv, Table 1.1) in 2023 was considered in the ‘total dose’ assessments, but this made an insignificant contribution to the highest ‘total dose’ in the vicinity of the Sellafield site.
The most significant contributors to the ‘total dose’ in the vicinity of the Sellafield site, 2023 were from crustacean consumption (98%), fish consumption (2%) and other pathways (<1%). The listed food groups are consumption pathways, the other pathways include external exposure over sediment. The most important radionuclide was polonium-210 (95%) with transuranic radionuclides (including plutonium-239+240 and americium-241) contributing less than 2% of the dose.
The dose in 2023 from artificial radionuclides discharged by Sellafield (including external radiation) and from historical discharges of naturally occurring radionuclides (from past non-nuclear industrial activity) contributed 0.008mSv and 0.21mSv, respectively. In 2022, the contributions were 0.014mSv and 0.22mSv, respectively. In 2023, the contribution from external radiation was 0.001mSv (0.002mSv in 2022). Data for naturally occurring radionuclides in fish and shellfish, and their variation in recent years, are discussed in Section 7.5.
The contribution to the ‘total dose’ of 0.008mSv in 2023 from artificial radionuclides (including external radiation) was lower, in comparison to that in 2022. The decrease in the contribution to the ‘total dose’ from 2023 was mostly attributed the update of habits information, in particular the reduction in consumption rates of fish and crustacean species. The contributing radionuclides in 2023 were mostly iodine-129 (35%), americium-241 (35%), plutonium radionuclides (6%) and other radionuclides (including carbon-14, caesium-137) (13%). External exposure contributed 11% of the ‘total dose’ from artificial radionuclides (20% in 2022).
Other age groups received less exposure than the adults ‘total dose’ of 0.22mSv in 2023 (10-year-old children: 0.12mSv; 1-year-old infants: 0.077mSv; prenatal children: 0.028mSv). ‘Total doses’ estimated for each age group may be compared with the dose for each person of approximately 2.3mSv to members of the UK population from exposure to natural radiation in the environment (Oatway and others, 2016) and to the annual dose limit to members of the public of 1mSv.
‘Total dose’ from gaseous discharges and direct radiation
In 2023, the dose to the representative person receiving the highest ‘total dose’, which includes contributions from artificial and naturally occurring radionuclides, from the pathways predominantly relating to gaseous discharges and direct radiation was 0.043mSv (Table 3.16). This is an increase from 0.011mSv in 2022 (as amended in the RIFE 28 errata). The most exposed age group in 2023 was an adult who consumed freshwater fish, who also consumed sea fish and spent time over intertidal substrates and a change from 2022. The increase in the ‘total dose’ and change in representative person was due to the revision of habits information. The most significant contributors in 2023 to the ‘total dose’ for adults were from external exposure over sediments (41%), consumption of sea water fish (39%), consumption of root vegetables (8%), and occupancy for direct radiation (9%). The most important radionuclides were iodine-129 (in seafood, 13%), polonium-210 (in seafood, 9%), lead-210 (in seafood, 12%), americium-241 (7%), carbon-14 (3%) and strontium-90 (<1%). External exposure contributed 0.020mSv (50%). Other age groups received lower exposure than the ‘total dose’ for adults of 0.043mSv (10-year-old children: 0.023mSv, 1-year-old infants: 0.012mSv and prenatal children: 0.028mSv).
Contributions to the highest annual ‘total dose’, from gaseous discharge and direct radiation sources and by specific radionuclides, are given in Figure 3.9 over the period 2012 to 2023. Over a longer period, the trend is of declining dose (Figure 2.9, (Environment Agency, Food Standards Agency, Food Standards Scotland, Natural Resources Wales, and others, 2018)) due to a general reduction in concentrations of radionuclides in food and the environment caused, in part, by reductions in discharges in this period. Over the period 2012 to 2019, ‘total doses’ were generally similar between years. The lower ‘total dose’ values after 2014 were mostly due to changes in the monitoring programme (Environment Agency and others, 2015). In 2018, the decrease in ‘total dose’ was mostly attributed to the revision of habits information following the full habits survey undertaken that year. From 2020, the relative changes in dose were largely due to variations in americium-241 concentrations (at limits of detection) in root vegetables. The increase in 2023, is due to the revision of the habits data and the majority of the dose (~80%) comes from aquatic pathways.
Figure 3.9. Contributions to ‘total dose’ from gaseous discharge and direct radiation sources at Sellafield, 2012 to 2023 (+ based on limits of detection for concentrations in foods).
‘Total dose’ from liquid discharges
The people receiving the highest ‘total dose’ from the pathways predominantly relating to liquid discharges are given in Table 3.16. Each ‘total dose’ is the same as that giving their maximum ‘total dose’ for all sources and pathways.
Source specific doses
Important source specific assessments of exposures, as a result of radioactive waste discharges from Sellafield, continued to be due to high-rate consumption of seafood and external exposure from gamma rays over long periods. Other pathways were kept under review, particularly the potential for sea to land transfer at the Ravenglass Estuary to the south of the site, exposure from contact with beta-emitting radionuclides during handling of sediments and/or handling of fishing gear and from gaseous discharges, the high-rate consumption of locally grown food.
Doses from terrestrial food consumption
In 2023, infants (1-year-old) consuming milk at high rates and exposed to external and inhalation pathways from gaseous discharges received the highest dose for all ages. The estimated dose was 0.012mSv in 2023 (Table 3.16), or approximately 1% of the dose limit to members of the public and up from 0.011mSv in 2022 (as corrected in the RIFE 28 errata). Other age groups received less exposure than the infants (1-year-old) dose of 0.010mSv in 2022 (adults: 0.010mSv; 10-year-old children: 0.009mSv; prenatal children: 0.005mSv).
Doses from seafood consumption
Two sets of habits data are used in these dose assessments. One is based on the habits information seen in the area each year (2023 habits survey). The second is based on a five-year rolling average using habits data gathered from 2019 to 2023. Some changes were found in the amounts (and mixes) of species consumed compared to those in the 2023 and the 2019 to 2023 rolling average. For crustaceans (crab, lobster, and other crustaceans), the total consumption rate decreased in 2023, and in the 2019 to 2023 rolling average. For fish (cod, other fish), the total consumption rate decreased in 2023 and in the 2019 to 2023 rolling average. For molluscs (winkles and other molluscs), the total consumption rates decreased in 2023 and in the 2018 to 2023 rolling average. The occupancy rate over sediments increased in the 2023 habits information and increased in the 2019 to 2023 rolling average. The revised habits data are given in Appendix 4 (Table A4.2).
Aquatic pathway habits are normally the most important in terms of dose near Sellafield and are surveyed every year (for example (Moore and others, 2023)). This allows generation of a unique yearly set of data and also rolling five-year averages. The rolling averages are intended to smooth the effects of sudden changes in habits and provide an assessment of dose that follows more closely changes in radioactivity concentrations in food and the environment. These are used for the main assessment of doses from liquid discharges and follow the recommendations of the report of the consultative exercise on dose assessments (CEDA) (Food Standards Agency 2001).
Table 3.16 summarises source specific doses to seafood consumers in 2023. The doses from artificial radionuclides to people who consume a large amount of seafood were 0.027mSv (0.027mSv in 2022) and 0.039mSv (0.045mSv in 2022) using the annual and five-year rolling average habits data, respectively. These doses each include a contribution due to external radiation exposure over sediments.
The dose to a local person (high-rate consumer of seafood), due to the enhancement of concentrations of naturally occurring radionuclides resulting from discharges from the former phosphate works near Whitehaven (using maximising assumptions for the dose coefficients and the five-year rolling average habits data), is estimated to have been 0.32mSv in 2023 and down from 0.33mSv in 2022. Most of this was due to polonium-210 (98%). For comparison (with the assessment using the five-year rolling average habits data), the dose from the single-year assessment for the Cumbrian coastal community seafood consumer from naturally occurring radionuclides (based on consumption rates and habits survey data in 2023) was 0.21mSv (Table 3.16).
Taking artificial and enhanced natural radionuclides together, the source specific doses were 0.23mSv and 0.36mSv for the annual and five-year rolling average habits data, respectively. These estimates are slightly higher or similar than the estimate of ‘total dose’ from all sources of 0.23mSv. The main reason for this is a difference in the approach to selecting consumption rates for seafood for the representative person. The differences in dose are expected and are within the uncertainties in the assessments (see Section 2.13).
Exposures typical of the wider communities associated with fisheries in Whitehaven, Dumfries and Galloway, the Morecambe Bay area, Northern Ireland and North Wales have been kept under review in 2023 (Table 3.15). Those for fisheries in the Isle of Man and Fleetwood have been shown to be generally lower and dose data are available in earlier RIFE reports (for example (Environment Agency, Food Standards Agency, Natural Resources Wales and others, 2014)). Where appropriate, the dose from consumption of seafood is summed with a contribution from external exposure over intertidal areas. The doses received in the wider communities were significantly lower than for the Cumbrian coastal community because of the lower concentrations and dose rates further afield. There were generally small changes in the doses (and contribution to doses) in each area in 2023 (Table 3.15), in comparison to those in 2022. For example, on the Dumfries and Galloway coast, the decrease in dose, in 2023, to 0.021mSv (from 0.024mSv in 2022) was mostly due lower gamma dose rates measured over different substrates. All annual doses of the wider communities were well within the dose limit for members of the public of 1mSv.
The dose to a person, who typically consumes 15kg of fish per year from landings at Whitehaven is also given in Table 3.16. This dose was less than 0.005mSv in 2023. The consumption rate used represents an average for a typical consumer of seafood from the north-east Irish Sea.
Doses from sediments
The main radiation exposure pathway associated with sediments is due to external dose from gamma-emitting radionuclides adsorbed on intertidal sediments in areas frequented by the public. This dose can make a significant contribution to the total exposure of members of the public in coastal communities of the north-east Irish Sea but particularly in Cumbria and Lancashire. Gamma dose rates currently observed in intertidal areas are mainly due to radiocaesium and naturally occurring radionuclides. For some people, the following pathways may also contribute to doses from sediments: exposure due to beta-emitting radionuclides during handling of sediments or fishing gear; inhalation of re-suspended beach sediments; and inadvertent ingestion of beach sediments. These pathways are considered later. In the main, they give rise to only minor doses compared with those due to external gamma-emitters.
Gamma radiation dose rates over areas of the Cumbrian coast and further afield in 2023 are given in Table 3.9. The results of the assessment of external exposure pathways are included in Table 3.16. The highest whole-body exposures due to external radiation resulting from Sellafield discharges, past and present, was received by a local houseboat dweller at Barrow, Cumbria. In 2023, the dose was 0.040mSv, or approximately 4% of the dose limit, and up from 0.029mSv in 2022 (see Section 6.2). Other people received lower external doses in 2023. The dose to a person who spends a long time over the marsh in the Ravenglass Estuary was 0.013mSv in 2023, and a decrease from that in 2022 (0.015mSv, as amended in the RIFE 28 errata). This decrease in dose was due to lower occupancy over salt marsh (Appendix 4, Table A4.2).
The doses to people in 2023 were also estimated for several other activities. Assessments were undertaken for a typical resident using local beaches for recreational purposes at 300 hours per year, and for a typical tourist visiting the coast of Cumbria with a beach occupancy of 30 hours per year. The exposure to residents was assessed for 2 different environments (at several locations) and at a distance from the Sellafield influence. The 2 different environments are 1) residents that visit and use beaches, and 2) residents that visit local muddy areas or salt marsh. Typical occupancy rates (Clyne and others, 2008; Clyne and others, 2010) are assumed and appropriate gamma dose rates have been used from Table 3.9. The activities for the typical tourist include consumption of local seafood and occupancy on beaches. Concentrations of radioactivity in fish and shellfish have been used from Table 3.5 to Table 3.7, and appropriate gamma dose rates used from Table 3.9. The consumption and occupancy rates for activities of a typical resident and tourist are provided in Appendix 4 (Table A4.2).
In 2023, the doses to people from recreational use of beaches varied from less than 0.005 to 0.009mSv (Table 3.16), with the higher doses being closer to the Sellafield source. The doses for recreational use of salt marsh and muddy areas had a similar variation, from less than 0.005 to 0.008mSv. The values for these activities were similar to those in recent years. The annual dose to a typical tourist visiting the coast of Cumbria, including a contribution from external exposure, was estimated to be less than 0.005mSv.
Doses from handling fishing gear and sediment
Exposures can also arise from contact with beta-emitting radionuclides during handling of sediments, or fishing gear on which fine particulates have become trapped. Habits surveys keep under review the amounts of time spent by fishermen handling their fishing gear, and by bait diggers and shellfish collectors handling sediment. For those most exposed, the rates for handling nets and pots and for handling sediments are provided in Appendix 4 (Table A4.2). In 2023, the skin doses to a bait digger and shellfish collector from handling sediment was 0.074mSv (Table 3.16). This was less than 0.5% of the appropriate annual dose limit of 50mSv specifically for skin. The skin dose to a fisherman from handling fishing gear (including a component due to naturally occurring radiation), based on 2019 monitoring data was 0.14mSv. Therefore, both handling of fishing gear and sediments continued to be minor pathways of radiation exposure.
Doses from atmospheric sea to land transfer
At Ravenglass, the representative person was infants (1-year-old) from consuming terrestrial foods that were potentially affected by radionuclides transported to land by sea spray. In 2023, the dose (including contributions from Chernobyl and fallout from nuclear weapons testing) was estimated to be 0.013mSv, which was approximately 1% of the dose limit for members of the public, and up from 0.011mSv in 2022 (as amended in the RIFE 28 errata). The increase in dose is attributed to higher carbon-14 concentrations in milk, and to a lesser extent domestic fruit, in 2023. The largest contribution to the dose was from ruthenium-106 in milk, as in recent years. As in previous years, sea-to-land transfer was not of radiological importance in the Ravenglass area.
Doses from seaweed and sea-washed pasture
Estimated annual doses for a high-rate consumer of laverbread (brown seaweed), and a high-rate consumer of vegetables (assuming these foods were obtained from the monitored plots near Sellafield and seaweeds were used as fertilisers and/or soil conditioners), are available in earlier RIFE reports (for example (Environment Agency, Food Standards Agency, Natural Resources Wales and others, 2014)). It has been previously established that the exposure pathway for a high-rate consumer of laverbread is of low radiological significance. No harvesting of Porphyra in west Cumbria, for consumption in the form of laverbread, was reported in the 2023 habits survey (Moore and others, 2024c) having been reported in the 2018 survey this exposure pathway has remained dormant in previous years. Previously reported doses from the consumption of vegetables using seaweed (as a fertiliser) have remained similar (and low) from year to year, with only minor variations in exposure (due to different foods being grown and sampled from the monitored plots). Exposures of vegetable consumers using seaweed from further afield in Northern Ireland, Scotland and North Wales are expected to be much lower than near Sellafield.
Animals may also graze on seaweeds on beaches in coastal areas. However, there has been no evidence of this taking place significantly near Sellafield. A research study (relevant to the Scottish islands and coastal communities) conducted by UKHSA on behalf of the FSA and SEPA, investigated the potential transfer of radionuclides from seaweed to meat products and also to crops grown on land where seaweed had been applied as a soil conditioner (Brown and others, 2009). The study concluded that the highest levels of dose to people using seaweed, as a soil conditioner or an animal feed, were in the range of a few microSieverts (µSv) and most of the doses are at least a factor of 100 lower. The report is available on SEPA’s website: SEPA environmental monitoring and assessment reports.
3.3.2 Gaseous discharges
Permitted discharges to atmosphere are made from a wide range of facilities at the site including the fuel storage ponds, the reprocessing plants and waste treatment plants, as well as from Calder Hall Power Station. Discharges from Calder Hall are now much reduced since the power station ceased generating electricity in 2003. Discharges to atmosphere, during 2023 are summarised in Appendix 1 (Table A1.1). The permit limits gaseous discharges for gross alpha and beta activities, and 8 specified radionuclides (the limits for krypton-85 and antimony-125 were removed in October 2023). In addition to overall site limits, plant notification levels have been set on discharges from the main contributing plants on site.
Discharges of gaseous wastes from Sellafield were much less than the permit limits in 2023. Gaseous discharges of ruthenium-106, caesium-137, plutonium-alpha and americium-241 and curium-242, increased in 2023, in comparison to releases in 2022.
Monitoring around the site related to gaseous discharges
Monitoring of terrestrial foods in the vicinity of Sellafield is conducted by the FSA to reflect the scale and risk of discharges from the site. This monitoring is the most extensive of that for the nuclear licensed sites in the UK. A range of foodstuffs was sampled in 2023 including milk, fruit, vegetables, meat and offal, game, and environmental materials (grass and soil). Samples were obtained from different locations around the site to allow for variations due to the influence of meteorological conditions on the dispersal of gaseous discharges. The analyses conducted included gamma-ray spectrometry and specific measurements for tritium, carbon-14, strontium-90, technetium-99, iodine-129, uranium and transuranic radionuclides.
The results of monitoring in 2023 are given in Table 3.4. The activity concentrations of all radionuclides around the site were low. Activity concentrations in terrestrial foodstuffs were generally similar to those in recent years. Activity concentrations of radionuclides in meat and offal (cattle and sheep) were low, with many reported as less than values with only very limited evidence of the effects of Sellafield’s gaseous discharges, detected in concentrations of carbon-14 in offal samples.
A range of foods (including fruit and vegetables) and terrestrial indicator materials was sampled in 2023, and the activity concentrations were generally similar to those found in previous years. In common with meat and offal samples, only limited evidence of the gaseous discharges from Sellafield was found in some of these foods. Strontium-90 was positively detected in a number of food samples (including milk) at low concentrations. As in 2023, the maximum iodine-129 and iodine-131 concentrations in milk were reported as less than values. Small enhancements (above the expected background) in concentrations of carbon-14 were found in some food samples (including milk), as in recent years. Concentrations of transuranic radionuclides, when detectable in these foods, were very low. Trends in maximum concentrations of radionuclides in milk (near Sellafield), and corresponding discharges of carbon-14, strontium-90 and caesium-137, for more than a decade are shown in Figure 3.10. Over the whole period, concentrations of carbon-14 were relatively constant (with some variation between years, generally consistent with changes in discharges), and caesium-137 concentrations (and strontium-90 to a lesser extent) were declining overall.
Figure 3.10. Discharges of gaseous wastes and monitoring of milk near Sellafield, 2012 to 2023.
3.3.3 Liquid discharges
Permitted liquid discharges derive from a variety of sources at the site including the fuel storage ponds, the reprocessing plants, from the retrieval and treatment of legacy wastes, the laundry and general site drainage. Wastes from these sources are treated and then discharged to the Irish Sea via the sea pipelines that terminate 2.1km beyond low water mark. Liquid wastes are also discharged from the factory sewer to the River Ehen Estuary and (since 2015) some liquid wastes are also discharged via the Calder Interceptor Sewer (Environment Agency, Food Standards Agency, Scotland, and others, 2016). Discharges from the Sellafield pipelines during 2023 are summarised in Appendix 1 (Table A1.2). The current permit sets limits on gross alpha and beta, and 12 individual radionuclides. In addition to overall site limits, plant notification levels have been set on discharges from the main contributing plants on site (Segregated Effluent Treatment Plant, Site Ion Exchange Effluent Plant (SIXEP), Enhanced Actinide Removal Plant (EARP) and THORP).
All discharges of liquid wastes from Sellafield were much less than the permit limits in 2023. Liquid discharges of all radionuclides decreased, by small amounts in 2023, in comparison to releases in 2022. To date, the discharges continue to reflect the varying amounts of fuel reprocessed in THORP (up to cessation in November 2018) and the Magnox reprocessing facility (which ceased in July 2022), and periods of planned and unplanned reprocessing plant shutdowns that occur from year to year.
The downward trend of technetium-99 discharges from Sellafield is given in Figure 3.11 (2012 to 2023) and Figure 3.12 (1994 to 2023). Technetium-99 discharges have substantially reduced from the peak of 192TBq in 1995. Further information relating to past discharges of technetium-99 is available in earlier RIFE reports (for example (Environment Agency, Food Standards Agency, Food Standards Scotland, Natural Resources Wales, and others, 2019)).
Figure 3.11. Technetium-99 in UK seaweed (‘Fucus vesiculosus’).
Figure 3.12. Technetium-99 in UK seaweed (‘Fucus vesiculosus’) from Sellafield liquid discharges between, 1994 to 2023.
Monitoring of the marine environment
Regular monitoring of the marine environment near to Sellafield and further afield was conducted during 2023, by the Environment Agency and FSA (for England and Wales), NIEA (for Northern Ireland) and SEPA (for Scotland). In Scotland, SEPA and the FSS work closely together to maximise the value of every sample taken as it is fully funded by the Scottish Government. The monitoring locations for seafood, water, environmental materials and dose rates near the Sellafield site are shown in Figure 3.13 and Figure 3.14, the corresponding sample species/types and locations are listed in the corresponding site tables.
Figure 3.13. Monitoring locations in Cumbria, 2023 (not including farms).
Figure 3.14. Monitoring locations at Sellafield, 2023 (not including farms).
Monitoring of fish and shellfish
Concentrations of beta/gamma activity in fish from the Irish Sea and from further afield are given in Table 3.5. Data are listed by location of sampling or landing point, north to south in Cumbria, then in approximate order of increasing distance from Sellafield. Results are available for previous specific surveys in the ‘Sellafield Coastal Area’ (extending 15km to the north and to the south of Sellafield, from St Bees Head to Selker, and 11km offshore) and the smaller ‘Sellafield Offshore Area’ (consisting of a rectangle, 1.8km wide by 3.6km long, situated south of the pipelines) in earlier RIFE reports (for example, (Environment Agency, Food Standards Agency, Natural Resources Wales and others and others,2014)). Concentrations of specific naturally occurring radionuclides in fish and shellfish in the Sellafield area are given in Section 7.
The concentrations of most radionuclides have decreased over the previous decades in response to decreases in discharges (for example Figure 2.8 to Figure 2.13, (Environment Agency and others, 2011)). Concentrations generally continue to reflect changes in discharges over time periods, characteristic of radionuclide mobility and organism uptake. More recent trends in concentrations of radionuclides, and corresponding discharges, in seafood near Sellafield (over the last decade) are shown in Figure 3.15 to Figure 3.20. There was variability from year to year, particularly for the more mobile radionuclides. Over the period 2012 to 2023, discharges and the concentrations of carbon-14 in fish and shellfish have declined (Figure 3.15). Discharges of cobalt-60 have decreased over the period 2012 to 2023 (Figure 3.16), however concentrations in fish and shellfish have shown some variation year on year, albeit at low concentrations. Liquid discharges of technetium-99 and concentrations of technetium-99 in fish and shellfish in 2023 (Figure 3.17) were similar, in comparison to their respective values in recent years. Over a longer timescale, technetium-99 concentrations in fish and shellfish have shown a continued reduction, from the relatively elevated values in the previous decade (for example Figure 2.10, (Environment Agency and others, 2011)). For the transuranic elements (Figure 3.19 and Figure 3.20), the trend of reductions in concentrations is not evident, unlike in earlier decades (for example Figure 2.12, (Environment Agency and others, 2011)). Over the last decade, discharges and concentrations of americium-241 and plutonium-239+240 in fish and shellfish have continued to show some variations from year to year (Figure 3.19 and Figure 3.20). Overall, these concentrations in shellfish have decreased over the period. The mean concentrations of carbon-14, cobalt-60, technetium-99, caesium-137, plutonium-239+240 and americium-241 in shellfish, were slightly lower in 2023, in comparison to those in 2022.
Figure 3.15. Carbon-14 liquid discharge from Sellafield and concentrations in plaice, lobsters and winkles near Sellafield, 2012 to 2023.
Figure 3.16. Cobalt-60 liquid discharge from Sellafield and concentrations in plaice, lobsters and winkles near Sellafield, 2012 to 2023.
Figure 3.17. Technetium-99 liquid discharge from Sellafield and concentrations in plaice, lobsters and winkles near Sellafield, 2012 to 2023.
Figure 3.18. Caesium-137 liquid discharge from Sellafield and concentrations in plaice, lobsters and winkles near Sellafield, 2012 to 2023.
Figure 3.19. Plutonium-239+240 liquid discharge from Sellafield and concentrations in plaice, lobsters and winkles near Sellafield, 2012 to 2023.
Figure 3.20. Americium-241 liquid discharge from Sellafield and concentrations in plaice, lobsters and winkles near Sellafield, 2012 to 2023.
Beta- and gamma-emitting radionuclides detected in fish included tritium, carbon-14, strontium-90 and caesium-137 (Table 3.5). Overall, concentrations of caesium-137 in fish species, across a wide range of sampling locations, were generally similar in 2023, in comparison to those in 2022. Over the longer time period, activity concentrations in fish and shellfish appear to be generally declining (with minor variations) at a slow rate (Figure 3.18). Activity concentrations in fish (and shellfish) generally reflected progressive dilution with increasing distance from Sellafield. However, the rate of decline of caesium-137 concentrations with distance was not as marked as was the case when significant reductions in discharges were achieved in earlier decades.
Other artificial beta- and gamma-emitting radionuclides detected in fish included carbon-14 and tritium (Table 3.5). With an expected carbon-14 concentration from natural sources of about 20Bq kg-1 (see Appendix 6, Table A6.1), the data suggest a continued local enhancement of carbon-14 due to discharges from Sellafield. In 2023, carbon-14 is reported as the highest activity concentration in marine fish (plaice, 45Bq kg-1) from Whitehaven. In 2023, the majority of both tritium and organically bound tritium (OBT) values, across all species and locations, were reported as less than values, with a value just above the LoD reported in Whitehaven plaice (27Bq kg-1). Promethium-147 was detected at a very low concentrations (reported as just above the less than value) in fish and shellfish in 2023.
For shellfish, a wide range of radionuclides is detectable, owing to generally greater uptake of radioactivity by these organisms from sediments. Generally, molluscs tend to contain higher concentrations than crustaceans and both contain higher concentrations than fish. Concentrations of beta- and gamma-emitting radionuclides are shown in Table 3.6 (Table 3.7 for plutonium-241). There can be substantial variations between species; for example, lobsters tend to concentrate more technetium-99 than crabs (as shown in references, (Knowles, Smith & Winpenny 1998; Swift & Nicholson 2001)). The highest concentrations in the marine environment from Sellafield discharges were carbon-14, tritium and technetium-99. Comparing 2022 and 2023 data across a wide range of sampling locations and shellfish species (where comparisons can be made), technetium-99 concentrations were similar (with minor variations) but reduced in comparison to those years prior to 2012 due to the progressive reductions in discharges of this radionuclide. Concentrations of other radionuclides (non-transuranic) in 2023 were also broadly similar (where comparisons can be made) to those in 2022.
Transuranic radionuclide data for fish and shellfish samples (chosen on the basis of potential radiological significance) in 2023 are given in Table 3.7. Transuranic elements are less mobile than other radionuclides in seawater and have a high affinity for sediments. This is reflected in higher concentrations of transuranic elements in shellfish compared with fish. Comparing 2023 and 2022 data across a wide range of sampling locations and shellfish species further afield from Sellafield, concentrations in shellfish were generally similar (where comparisons can be made). Those from the north-eastern Irish Sea were the highest transuranic concentrations found in foodstuffs in the UK. In 2023, the concentrations of plutonium and americium-241 in shellfish were generally higher (by small amounts) in comparison to those in 2022 at most of the north-eastern Irish Sea locations (for example, winkles from Nethertown and Parton). Americium-241 concentrations in mussels (near Sellafield) were also generally similar in 2023, in comparison to those in 2022. Overall, plutonium-239+240 and americium-241 concentrations in lobsters (near Sellafield) were generally lower (with minor variations) in 2023, in comparison to those in recent years. The concentrations of plutonium-239+240 and americium-241 in winkles (Nethertown) and lobster (Sellafield Coastal Area) in 2023 were the lowest reported values in recent years (Figure 3.19 and Figure 3.20). Variations of these observations in previous years were likely to have resulted from a combination of mechanisms including natural environmental variability and redistribution of sediments due to natural processes.
Monitoring of sediments
Radionuclides in Sellafield liquid discharges are taken up into sediments along the Cumbrian Coast, in particular in muddier (fine grained) areas such as estuaries. Some of these areas are used by the public. Concentrations of radionuclides are regularly monitored, both because of their relevance to exposure and to keep distributions of radioactivity under review. The results for 2023 are shown in Table 3.8. Radionuclides positively detected were cobalt-60, strontium-90, caesium-137, europium-154, europium-155, and transuranic elements. The highest concentrations found are close to the site and in fine particulate materials in estuaries and harbours, rather than the coarser grained sands on open beaches. In 2023, the concentrations of caesium-137 and plutonium radionuclides were higher in the River Mite Estuary (an erosional area), in comparison to those in 2022. The concentrations of long-lived radionuclides, particularly caesium-137 and the transuranic elements, largely reflect past discharges from Sellafield, which were considerably higher than in recent years. Over the last 4 decades, discharges have fallen significantly as the site provided enhanced treatment to remove radionuclides prior to discharge. Overall, concentrations in sediments were generally similar in 2023, in comparison to those in 2022.
The trends over time (1994 to 2023) for activity concentrations in mud from Ravenglass and liquid discharges from Sellafield are shown in Figure 3.21 to Figure 3.24. The concentrations of most radionuclides have declined over the time period in response to decreases in discharges, with sustained reductions in discharges of caesium-137 and transuranic elements. Discharges of cobalt-60 have been variable in the earlier years but reduced over the last decade, as reflected in the sediment concentrations at Ravenglass, with some evidence of a lag time between discharge and sediment concentration (Figure 3.23). In 2022, the reported cobalt-60 concentration in mud from Ravenglass (Newbiggin) is the lowest reported value in recent years. Over the last decade, caesium-137 and transuranic concentrations in sediments have remained relatively constant (Figure 3.21, Figure 3.22 and Figure 3.24). Since the mid-1990s, discharges of caesium-137, plutonium isotopes and americium-241 have remained low, but with some variability. There is a suggestion of small progressive increases in caesium-137 and transuranic elements activities in sediments (peaking in both 2006 and 2014). The likely explanation is that changes in these concentrations are due to remobilisation and subsequent accretion of fine-grained sediments containing higher activity concentrations. For americium-241, there is also an additional contribution due to radioactive in-growth from the parent plutonium-241 already present in the environment. The effect is less apparent in fish and shellfish (Figure 3.18 to Figure 3.20) and will continue to be monitored.
Figure 3.21. Caesium-137 liquid discharge from Sellafield and concentration in mud at Ravenglass, 1994 to 2023.
Figure 3.22. Plutonium-alpha liquid discharge from Sellafield and plutonium-239+240 concentration in mud at Ravenglass, 1993 to 2023.
Figure 3.23. Cobalt-60 liquid discharge from Sellafield and concentration in mud at Ravenglass, 1994 to 2023.
Figure 3.24. Americium-241 liquid discharge from Sellafield and concentration in mud at Ravenglass, 1994 to 2023.
Concentrations of caesium-137 and americium-241 in sediments from coastal locations of the north-east Irish Sea, including locations on the Dumfries and Galloway coast, Cumbrian coastline, Flookburgh, River Ribble estuary and North Wales coast, are also shown in Figure 3.25. Concentrations of both radionuclides diminish with distance from Sellafield. Overall, concentrations in 2023 at a given location were generally similar to those in recent years, and any fluctuations were most likely due to the normal variability expected to be in the environment.
Figure 3.25. Concentrations of americium-241 and caesium-137 in coastal sediments in Northwest England, North Wales and Southwest Scotland between 1999 to 2023 (Note different scales used for Newbiggin and Carleton Marsh).
Monitoring of dose rates
Dose rates are regularly monitored at many locations, both in the Sellafield vicinity and further afield, using environmental radiation dosimeters. Table 3.9 provides the locations monitored by the environment agencies and the gamma dose rates in air at 1m above ground. Where comparisons can be made from similar ground types and locations, dose rates over intertidal areas throughout the Irish Sea in 2023 were generally similar to those in recent years (with small variations in comparison to those in 2022). Any variations between years are likely to have been due to normal variability expected to be present in the environment. As in previous years, gamma dose rates were measured on the banks of the River Calder, which flows through the Sellafield site. In 2023, gamma dose rates did not show a significant excess above natural background downstream of the site. Although these dose rates have been locally enhanced in previous years on the banks of the River Calder, occupancy by the public (mainly anglers) is low in this area, this is unlikely to be more than a few tens of hours per year. On this basis, the resulting doses (in previous years) were also much less than those at other intertidal areas as discussed earlier in this section.
Gamma dose rates above mud and salt marshes, from a range of coastal locations in the vicinity of Sellafield, are shown in Figure 3.26 (2012 to 2023). Gamma dose rates at sandy locations are generally lower than those above mud or salt marshes. The general decrease in dose rates with increasing distance from Sellafield, which was apparent under conditions of higher discharges several decades ago, is no longer so prominent in recent years. Spatial variability of dose rates is expected, depending on ground type, with generally higher dose rates recorded over areas with finely divided sediments. For each location, there has been variation over time. Close to Sellafield (at Carleton Marsh and Newbiggin), there is some evidence to suggest that dose rates were slowly declining over the time period. Locations that are further afield from Sellafield show dose rate values that only marginally exceeded average UK natural background rates.
Figure 3.26. Gamma dose rates above fine coastal sediments (mud and salt marshes) in Northwest England, North Wales and Southwest Scotland between 2012 to 2023.
Over the last 4 decades, concentrations of radioactivity in the environment around Sellafield have declined as a result of reduced discharges. In more recent years, the values in the Esk Estuary have shown a less clear trend, with concentrations of some radionuclides fluctuating from year to year (for example, see Figure 3.22). This effect could be due to the dynamic nature of the sediment in the estuary, which is eroded and transported by tide and freshwater, periodically exposing older sediment (from depth) containing radioactivity from historical discharges. Due to annual variations and local concerns, the Environment Agency initiated a more detailed study of dose rates in the Esk Estuary in 2007. Further information providing more background information, and describing the objectives and results of this study, is available in earlier RIFE reports (for example (Environment Agency and others, 2015)).
Monitoring of fishing gear
During immersion in seawater, fishing gear may trap particles of sediment on which radioactivity is adsorbed. Fishermen handling this gear may be exposed to external radiation, mainly to skin from beta particles. Up to 2019, fishing gear was regularly monitored using surface contamination monitors. As in 2022, no monitoring of fishing gear was performed in 2023. Results up to 2019 are included in previous RIFE reports (for example, (Environment Agency and others, 2020)).
Contact dose-rate monitoring of intertidal areas
Results from measurements of beta dose rates on shoreline sediments (using contamination monitors), to allow estimation of exposure of people who handle sediments regularly, are given in Table 3.10. Overall, positively detected dose rates in 2023 were generally similar to those in 2022 (where comparisons can be made from similar ground types and locations). Beta dose rates in sand were higher at St Bees in comparison to those in 2022. However, reported beta dose rates are low, with no radiological significance.
More general beta/gamma monitoring for the Environment Agency of radiological contamination on beaches using portable probes continued to establish whether there are any localised ‘hot spots’ of activity, particularly in strand lines and beach debris. In 2023, no material was found using these probes in excess of the action level equivalent to 0.01mSv h-1.
In 2008, the Environment Agency published a formal programme of work for the assessment of contamination by radioactive particles and objects[footnote 15] on and around the west Cumbrian coastline. The assessment was focused on public protection from high activity discrete radioactive particles that have been released to the environment from activities at the Sellafield site (Environment Agency 2008).
Beach survey work using vehicle mounted detectors, by the Sellafield site operator’s contractors, began in 2006. The current detection system in use is the Groundhog™ Synergy2 system, which was introduced in mid-2014 and was designed, and introduced, to further improve detection of americium-241 and strontium-90/yttrium-90. This replaced the Groundhog-TM Synergy system, which was used from mid-2009 to mid-2014, which had a specific capability in relation to the detection of medium/high energy gamma-emitting radionuclides. The GroundhogTM Synergy system also provided improved detection capability for low energy gamma emissions (in comparison to the original system introduced in 2006), increasing the ability to detect particles containing americium-241.
Further beach monitoring for the 2023 calendar year was completed in line with the Environment Agency’s specification. During 2023, a total area of 120 hectares of the beaches along the Cumbrian were surveyed against a programme target of 105 hectares. A total of 78 particles and 3 larger objects were detected, recovered and analysed. In 2017, there was a change implemented to the beach finds categories in that the ‘stone’ category is replaced by ‘larger object’. This means that all items larger than 2mm in size (for example granules, gravel, wire, pebbles, and stones) are now classified as objects. The number of radioactive finds identified was 81 in 2023, of which approximately 96% were classified as particles (less than 2mm in size) and the remainder as larger objects. The number of finds were typical of those in recent years. Most of the finds were concentrated on a 5km stretch of beach running northwest from the Sellafield site. All have been removed from the beaches. In 2023, none of the finds detected exceeded the characterisation triggers set within the Environment Agency’s intervention trigger levels: Sellafield radioactive objects intervention plan.
Monitoring along the Cumbrian coast will continue for 2024, with the current proposal being a further 105 hectares to be surveyed.
In 2012, UKHSA reported their review of the results and position on risk following the introduction of the improved monitoring (Groundhog™ Synergy system). The report concluded that the increase in particle finds following the introduction of this system was a result of its improved capability and also that advice previously given by UKHSA to the Environment Agency following a detailed assessment of risks in 2010 remained valid (Brown & Etherington 2011; Etherington and others, 2012). The report restated the conclusion that, based on the currently available information, the overall health risks to beach users are very low and significantly lower than other risks people accept when using the beaches. As such, UKHSA advice remained that no special precautionary actions were required to limit access to or use of the beaches. A report by UKHSA describes the assessed health risks from the consumption of seafood (including those to commercial fishermen) from radioactive particles in the vicinity of the Sellafield Site (Oatway & Brown 2015). Based on currently available information, it is concluded that the overall health risks to both seafood consumers and commercial fishermen are very low. More recently, UKHSA were requested by the Environment Agency to update their recommendations, if supported by available evidence. This is to account for the information from the beach monitoring programme and from the further analysis of finds that have been collected since 2012. A summary report of assessing the risk to people’s health from radioactive objects on beaches around the Sellafield site was published by UKHSA in February 2020, concluding that the risk is very low (Oatway and others, 2020).
In relation to food safety (and following a previous assessment of the particles frequency and the activity concentrations), FSA’s guidance to the Environment Agency supported UKHSA’s advice. The Environment Agency will continue to work with relevant authorities to keep the situation under review.
In 2007, SEPA published a strategy document for the assessment of the potential impact of Sellafield radioactive particles on members of the public in south-west Scotland (Scottish Environment Protection Agency 2007) and the beach monitoring programme was temporarily extended to include 2 locations on the north Solway coastline (Kirkcudbright Bay and Southerness). This was based on some limited modelling work on the movement of particles undertaken for the Environment Agency following a request by SEPA. No particles were detected at these locations. SEPA is maintaining a watching brief on the situation in as much as it may affect Scotland.
Further detail on enhanced beach monitoring data compiled so far can be obtained on the UK government website: Sellafield radioactive objects intervention plan - monitoring beaches near sellafield.
Monitoring of seaweed
Seaweeds are useful indicator materials, in addition to their occasional use in foods and as fertilisers. Seaweeds have the capability to readily accumulate radionuclides and thereby assist in the detection of these radionuclides in the environment. Table 3.11 gives the results of measurements in 2023 of seaweeds from shorelines of the Cumbrian coast and further afield. Comparing 2022 and 2023 data across a wide range of sampling locations, radionuclide concentrations were generally similar (where comparisons can be made) in seaweeds.
Fucus species of seaweeds are particularly useful indicators of most fission product radionuclides. In particular, samples of ‘Fucus vesiculosus’ are collected both in the Sellafield vicinity and further afield to show the extent of Sellafield contamination in north European waters. The effects of technetium-99 discharges from Sellafield on concentrations in seaweed are shown in Figure 3.11 (2012 to 2023) and Figure 3.12 (1994 to 2023). In the north-east Irish Sea, technetium-99 concentrations have been reasonably constant over the present decade, consistent with the relatively low discharges; the highest concentrations which were found near Sellafield were much less than those in the mid-1990s and the decade thereafter (in response to the progressive reduction in discharges). In general, there was also a large reduction in concentrations of technetium-99 in ‘Fucus vesiculosus’ with distance from Sellafield, as the effect of the discharges becomes diluted in moving further afield.
Technetium-99 concentrations in seaweed (Table 3.11) collected from sites in Cumbria were generally lower by small amounts in 2023, in comparison to those in 2022. Over the last 5 years, small variations have been found, year on year, but technetium-99 concentrations in seaweed in 2023 were still low (Figure 3.11). At one specific location (Auchencairn, Scotland), known to have had fluctuating concentrations in previous years, technetium-99 concentrations in seaweed (Fucus) were higher in 2023 compared with those in 2022. The reasons behind these variations have been described in previous RIFE reports (for example (Environment Agency, Food Standards Agency, Food Standards Scotland, Natural Resources Wales and others,2019)).
Monitoring of tide-washed pasture
The potential transfer of technetium-99 to milk, meat and offal from animals grazing tide-washed pasture was considered using a modelling approach in the report for 1997 (Ministry of Agriculture Fisheries and Food & Scottish Environment Protection Agency 1998). The maximum potential dose was calculated to be 0.009mSv per year, at that time. Follow-up sampling of tide-washed pastures at Newton Arlosh (Cumbria) and Hutton Marsh (Lancashire) in 2006 suggested that this dose estimate remains valid (Environment Agency and others, 2007).
Monitoring of sea to land transfer
Terrestrial foodstuffs are monitored near Ravenglass to check on the extent of transfer of radionuclides from sea to land in this area. In 2023, samples of milk and livestock were collected and analysed, for radionuclides which were released in liquid effluent discharges from Sellafield. Results from surveys for activity concentrations in crops, fruit and environmental indicators are available in earlier RIFE reports (for example, (Environment Agency, Food Standards Agency, Natural Resources Wales and others, 2014)).
The results of measurements in 2023 are given in Table 3.12. Generally, the activity concentrations, where positively detected, show lower concentrations than were found in the immediate vicinity of Sellafield (Table 3.4). As in previous years, the evidence for sea to land transfer was very limited in 2023. Technetium-99 concentrations are reported as less than values (or close to the less than value). Small concentrations of artificial nuclides were detected in some samples, but the concentrations were very low. As in recent years, where detectable, observed isotopic ratios of plutonium-238 to plutonium-239+240 concentrations were somewhat higher than 0.025, a ratio which might be expected if the source was entirely due to fallout from nuclear weapons testing. This may suggest a Sellafield influence.
Monitoring of fishmeal
A theoretical study has established that any indirect onward transmission of both naturally occurring and artificial radioactivity into the human diet from the fishmeal pathway (that is fed to farmed fish, poultry, pigs, cows and sheep) is unlikely to be of radiological significance (Smith & Jeffs 1999). A detailed survey was undertaken to confirm these findings (Food Standards Agency 2003). Samples, obtained from 14 fish farms in Scotland and 3 in Northern Ireland, contained very low radionuclide concentrations, most being less than the limits of detection, and the few positively detected values were all less than 1Bq kg-1. Annually reported RIFE results for activity concentrations in farmed salmon from the west of Scotland confirm the findings of the FSA study (for example, Tables 2.5 and 2.7 (Environment Agency, Food Standards Agency, Natural Resources Wales and others, 2014)).
Monitoring of waters
Evidence of the effects of liquid discharges from Sellafield on concentrations of radionuclides in seawater is determined by sampling from research vessels and the shore. The results of the seawater programme are given in Section 8.
Sampling of freshwater from rivers and lakes in west Cumbria is conducted as part of the regular environmental monitoring programme around Sellafield. However, other environmental materials are likely to be more indicative of direct site-related effects. Some of the sources monitored provide public drinking water. The results for 2023 are included in Table 3.13. Tritium, gross alpha and gross beta concentrations in public supplies were below the investigation levels for drinking water in the Water Supply (Water Quality) (Amendment) 2018 Regulations (retained from the European Directive 2013/51).
Small amounts of radioactivity are discharged from Sellafield under permit via the factory sewer outfall to the River Ehen Estuary, immediately prior to the confluence with the River Calder. In 2023, there was no evidence of tritium downstream or upstream of the outfall (Table 3.13). These are not drinkable waters, and any low concentrations observed previously are of no radiological significance. Table 3.13 also includes the results of monitoring from Ehen Spit beach (Figure 3.13) near Sellafield where water issues from the ground at low tide. This release is not due to permitted discharges of liquid wastes but to ground water migration from the Sellafield site. The water contains high levels of salt so it will not be used as a drinking water source and therefore the only consumption would be inadvertent (incidental). Enhanced gross beta and tritium concentrations were observed in 2023 with concentrations similar to those in recent years. The annual dose from inadvertent consumption of water from Ehen Spit has been shown to be insignificant (Environment Agency 2002).
Monitoring of unusual pathways
In 1998, high caesium-137 concentrations (up to 110,000Bq kg-1) were found in feral pigeons sampled in Seascale by MAFF (Ministry of Agriculture Fisheries and Food & Scottish Environment Protection Agency 1999). Further background information, describing the consequences of this monitoring, and remedial measures taken by the site operator, is available in earlier RIFE reports (for example (Environment Agency and others, 2015)). Like in 2022, wood pigeon was not sampled in 2023. In 2020, the maximum caesium-137 concentration in the muscle of wood pigeon was detected just above the less than value (0.49Bq kg-1) and generally similar to those in recent years. These caesium-137 concentrations fluctuated in value prior to 2011, but elevated concentrations have not been sustained thereafter. Concentrations of artificial radionuclides were low and would add little to the exposure of local consumers. The FSA will continue to monitor this pathway.
Following discovery of elevated concentrations in feral pigeons, the Environment Agency began to sample and analyse sediments from road drains (gully pots) in Seascale and Whitehaven in 1999. Gully pots in road drains collect sediments washed off road surfaces and provide good indicators of radiological contamination of urban environments. The results of analyses in 2023 are shown in Table 3.14. Overall, activity concentrations are generally similar to those in recent years, although plutonium-239+240 and americium-241 concentrations decreased, by small amounts, in 2023. Further information of the previously elevated concentrations (of strontium-90, caesium-137, americium-241 and plutonium radionuclides) in road drain sediments is given in earlier RIFE reports (for example (Environment Agency, Food Standards Agency, Food Standards Scotland, Natural Resources Wales, and others, 2019)).
4 Nuclear power stations
Highlights
- ‘total doses’ for the representative person were approximately 3% of the dose limit for all sites assessed
Operating sites
Hartlepool, County Durham
- ‘total dose’ for the representative person was 0.015mSv and increased in 2023
- gaseous discharges of carbon-14 increased in 2023
- liquid discharges of tritium, sulphur-35 and other radionuclides decreased in 2023
Heysham, Lancashire
- ‘total dose’ for the representative person was 0.015mSv and decreased in 2023
- liquid discharges of tritium from Heysham 1 decreased in 2023
Hinkley Point, Somerset
- ‘total dose’ for the representative person was 0.032mSv and increased in 2023
- gaseous discharges of carbon-14, sulphur-35 and argon-41 from Hinkley B decreased in 2023
- liquid discharges of tritium from Hinkley B decreased in 2023
Hunterston, North Ayrshire
- ‘total dose’ for the representative person was 0.013mSv and increased in 2023
- gaseous discharges of argon-41 were reported as nil from Hunterston B in 2023
- liquid discharges of plutonium-241 from Hunterston A were reported as nil and discharges of tritium and sulphur-35 from Hunterston B decreased in 2023
Sizewell, Suffolk
- ‘total dose’ for the representative person was less than 0.010mSv and decreased in 2023
- gaseous discharges carbon-14 from Sizewell B decreased in 2023
Torness, East Lothian
- ‘total dose’ for the representative person was 0.007mSv and increased in 2023
Decommissioning sites
Berkeley, Gloucestershire and Oldbury, South Gloucestershire
- ‘total dose’ for the representative person was less than 0.005mSv and unchanged in 2023 (see RIFE 28 Errata)
- gaseous discharges of carbon-14 increased in 2023
Bradwell, Essex
- ‘total dose’ for the representative person was less than 0.005mSv and unchanged in 2023
Chapelcross, Dumfries and Galloway
- ‘total dose’ for the representative person was 0.010mSv and increased in 2023
Dungeness, Kent
- ‘total dose’ for the representative person was 0.005mSv and decreased in 2023
Trawsfynydd, Gwynedd
- ‘total dose’ for the representative person was 0.007mSv and decreased in 2023
Wylfa, Isle of Anglesey
- ‘total dose’ for the representative person was less than 0.005mSv and decreased in 2023
- gaseous discharges of tritium and carbon-14 decreased in 2023
This section considers the results of environment and food monitoring, under the responsibility of the Environment Agency, FSA, FSS, NRW and SEPA, undertaken near nuclear power stations (both operating stations and decommissioning sites). There are a total of 19 nuclear power stations (which may contain more than 1 reactor and reactor type) at 14 locations in the UK as listed below:
- England
- Berkeley, Oldbury, Bradwell, Calder Hall, Dungeness, Hartlepool, Heysham, Hinkley Point and Sizewell
- Scotland
- Chapelcross, Hunterston and Torness
- Wales
- Trawsfynydd and Wylfa
Eleven of the 19 nuclear power stations are older, first generation, Magnox power stations, owned by the NDA. The NDA was set up under the Energy Act 2004 and is a non-departmental public body (sponsored and funded by DESNZ). Its remit is to secure the decommissioning and clean-up of the UK’s civil public sector nuclear licensed sites. All Magnox stations are now in the process of decommissioning.
All of the first-generation nuclear reactors are now fuel free. In April 2024, the NDA published its annual business plan (2024 to 2027) and a new strategy. The plan summarises the programme of work at each of the sites (Nuclear Decommissioning Agency 2024).
In 2013, Magnox Limited managed 10 nuclear sites and was owned and operated by Energy Solutions on behalf of the NDA. In 2014, the NDA formally appointed Cavendish Fluor Partnership (a joint venture between Cavendish Nuclear and Fluor Corporation) as the PBO for Magnox Limited and Research Sites Restoration Limited (RSRL). Thereafter, the 10 Magnox sites were re-licensed into a single site licensed company alongside the Harwell and Winfrith sites. In 2015, Harwell and Winfrith sites, previously operated by RSRL, merged to be part of Magnox Limited. In 2019, Magnox Limited became a wholly owned subsidiary of the NDA, replacing the previous PBO management model of ownership by the private sector. In April 2023, Dounreay joined with Magnox Limited (see more information in Section 5). In October 2023, Magnox Limited changed its brand and became Nuclear Restoration Services Limited (NRS Ltd). Calder Hall is being decommissioned, it is operated by Sellafield Limited and discharges from this Magnox power station are considered in Section 3 as it is located at Sellafield.
Seven AGR power stations and one PWR power station are owned and operated by EDF Energy Nuclear Generation Limited in 2023 these are: Dungeness B, Hartlepool, Heysham 1 and 2, Hinkley Point B, Sizewell B Power Stations in England, and Hunterston B and Torness Power Stations in Scotland. In 2021, EDF decided to move Dungeness B into defueling phase following an extended outage in the previous years. In June 2021, the UK government and EDF signed an agreement to transfer control of the AGR power stations to the NDA after cessation of generation and defueling of the reactors. Hunterston B and Hinkley Point B stopped generating electricity in January and August 2022, respectively. The decommissioning process of these two plants will be carried out by NRS after used fuel has been fully removed by EDF.
Gaseous and liquid discharges from each of the power stations are regulated by the Environment Agency and NRW in England and Wales, respectively and by SEPA in Scotland. In 2023, gaseous and liquid discharges were below regulated limits for each of the power stations (see Appendix 1, Table A1.1 and Table A1.2). Solid waste transfers in 2023 from nuclear establishments in Scotland (Chapelcross, Hunterston A, Hunterston B and Torness) are also given in Appendix 1 (Table A1.4). Independent monitoring of the environment around each of the power stations is conducted by the FSA and the Environment Agency in England and Wales, and by SEPA in Scotland. In Wales, this is conducted on behalf of NRW and the Welsh Government. Data tables and the detailed results of the dose assessments, in Open Document Spreadsheet (ODS) format, are downloadable from the main RIFE page
In this section, sites are grouped according to their status of power generation (operating and decommissioning power stations). With both an operating and decommissioning power stations, the Sizewell site was considered under operating sites. As the reactors at both Hunterston B and Hinkley Point B are still being defueled, both sites were also retained under the operating sites section. Therefore, Hartlepool, Heysham (1 and 2), Hinkley Point (A and B), Hunterston (A and B), Sizewell (A and B) and Torness are included under operating power stations (Section 4.1). Berkeley, Bradwell, Chapelcross, Dungeness (A and B), Oldbury, Trawsfynydd and Wylfa are included under decommissioning power stations (Section 4.2).
4.1 Operating sites
4.1.1 Hartlepool, County Durham
Hartlepool Power Station is situated on the mouth of the Tees Estuary, on the north-east coast of England. This station, which is powered by twin AGRs, began operation in 1983. In March 2023, EDF decided to extend the generating life of the Hartlepool power station by 24 months until March 2026. The most recent habits survey was undertaken in 2014 (Garrod, Clyne & Papworth 2015).
Doses to the public
The ‘total dose’ from all pathways and sources of radiation was 0.015mSv in 2023 (Table 4.1), which was under 2% of the dose limit to members of the public. This is an increase from 0.011mSv in 2022. The increase in ‘total dose’ was mainly attributed to a higher estimate of direct radiation from the site, in comparison to that in 2022. The representative person was an adult spending time over sand and sea coal and unchanged from 2022. The trend in ‘total dose’ over the period 2012 to 2023 is given in Figure 4,1. ‘Total doses’ remained broadly similar, from year to year, and were low.
Figure 4.1. ‘Total dose’ at Hartlepool nuclear power station, 2012 to 2023.
Year | Dose, mSv y-1 |
---|---|
Dose limit | 1 .0 |
2012 | 0.015 |
2013 | 0.024 |
2014 | 0.027 |
2015 | 0.022 |
2016 | 0.020 |
2017 | 0.031 |
2018 | 0.012 |
2019 | 0.013 |
2020 | 0.017 |
2021 | 0.012 |
2022 | 0.011 |
2023 | 0.015 |
A source specific assessment for high-rate consumers of locally grown foodstuffs gave an exposure that was less than the ‘total dose’ in 2023 (Table 4.1). As in 2022, the estimated dose was 0.005mSv. The dose to a local fish and shellfish consumer (including external radiation but excluding naturally occurring radionuclides) was 0.015mSv in 2023, and up from 0.013mSv in 2022. The reason for the increase in dose was mostly due to higher gamma dose rates measured over sand at Carr House in 2023.
As in recent years, a source specific assessment was not undertaken to determine the exposure from naturally occurring radionuclides in 2023, as a consequence of reported polonium-210 concentrations in mollusc samples. Prior to 2019, winkle samples collected from South Gare (inside the Tees Estuary entrance) also included some winkles taken from the estuary entrance near Paddy’s Hole. The area near Paddy’s Hole is polluted with oil and other wastes and therefore a potential reason for enhanced naturally occurring radionuclides in molluscs. As in recent years, due to limited availability, a winkle sample was not collected from Paddy’s Hole in 2023. This estimate assumes that the median concentrations for naturally occurring radionuclides at background (Appendix 6, Table A6.1) be subtracted from the total concentrations as measured in 2023.
Gaseous discharges and terrestrial monitoring
Gaseous radioactive waste is discharged via stacks to the local environment. Gaseous discharges of carbon-14 increased in 2023, in comparison with 2022, due to the increased number of times the reactors were de-pressurised. Analyses of tritium, carbon-14, sulphur-35 and gamma-emitting radionuclides were carried out in milk and crop samples. Samples of freshwater were also taken from boreholes. Data for 2023 are given in Table 4.2(a). The effects of gaseous disposals from the site were not easily detectable in foodstuffs, although a small enhancement of carbon-14 concentrations was measured in food (barley and potato) in 2023. As in recent years, carbon-14 was also detected in locally produced milk at concentrations slightly above the default value used to represent background. Tritium, and gross beta concentrations in freshwater were below the investigation levels for drinking water in the Water Supply (Water Quality) (Amendment) 2018 Regulations (retained from the European Directive 2013/51).
Liquid waste discharges and aquatic monitoring
Permitted discharges of radioactive liquid effluent are made to Hartlepool Bay with non-radioactive trade effluents being discharged directly to the River Tees. Liquid discharges in 2023 were broadly similar, in comparison with those in 2022.
Results of the aquatic monitoring programme conducted in 2023 are shown in Table 4.2(a) and Table 4.2(b). As in 2022, the carbon-14 concentration measured in the mollusc samples (winkles) were not above the expected background (Table A6.1). Carbon-14 concentrations in fish and crustaceans were generally similar in 2023, in comparison to those in recent years.
The analysis of technetium-99 in seaweed is used as a specific indication of the far-field effects of disposals to sea from Sellafield. As in recent years, technetium-99 in seaweed was low and much less than the peak concentration observed in 2008 (see Figure 2.11, (Environment Agency, Food Standards Agency, Food Standards Scotland, Natural Resources Wales and others, 2019)). Technetium-99 concentrations in seaweed are less than 1% of the equivalent concentrations near Sellafield.
As in recent years, apart from 2020, iodine-131 was positively detected in seaweed samples collected around the mouth of the River Tees Estuary in 2023. The detected values, as in previous years, are believed to originate from the therapeutic use of this radionuclide in local hospitals. Detectable concentrations of caesium-137 were mainly due to disposals from Sellafield and fallout from nuclear weapons testing. However, caesium-137 concentrations in sediment have remained low for several years (Figure 4.2). Overall, gamma dose rates over intertidal sediment in 2023 were slightly higher (where comparisons can be made from similar ground types and locations), to those observed in 2022.
Figure 4.2. Caesium-137 concentration in marine sediments near Hartlepool nuclear power station between 2012 to 2023. Data are presented to 2 significant figures.
Year | 137Cs concentration Bq kg-1 (dry) |
---|---|
2012 | 2.2 |
2013 | 2.4 |
2014 | 1.8 |
2015 | 1.4 |
2016 | 1.2 |
2017 | 1.3 |
2018 | 1.1 |
2019 | 1.5 |
2020 | 1.0 |
2021 | 0.91 |
2022 | 0.70 |
2023 | 0.76 |
In 2023, the reported polonium-210 and lead-210 concentrations in winkles from South Gare are values expected due to naturally occurring sources (given in Appendix 6, Table A6.1). As in recent years, a winkle sample could not be collected from the estuary entrance near Paddy’s Hole in 2023.
4.1.2 Heysham, Lancashire
Heysham Power Station is situated on the Lancashire coast, to the south of Morecambe and near the port of Heysham. This establishment comprises of 2 separate nuclear power stations, Heysham 1 and Heysham 2, each powered by twin AGRs. Heysham 1 commenced operation in 1983 and Heysham 2 began operating in 1988. It is estimated that Heysham 1 and 2 will continue to generate electricity until 2026 and 2028, respectively. Disposals of radioactive waste from both stations are permitted via separate and adjacent outfalls to Morecambe Bay and via stacks. However, in RIFE, both stations are considered together for purposes of environmental monitoring, because the discharges from both sites impact on the same area.
The most recent habits survey was conducted in 2016 (Garrod and others, 2017).
Doses to the public
The ‘total dose’ from all pathways and sources of radiation was 0.015mSv in 2023 (Table 4.1) or less than 2% of the dose limit for members of the public. This is a decrease from 0.016mSv in 2022. As in recent years, the representative person was an adult spending time over sediments. The small decrease in ‘total dose’ in 2023 was mostly due to lower gamma dose rates measured over sand in comparison to those in 2022.
The trend in ‘total dose’ over the period 2012 to 2023 is given in Figure 4.3. Between 2012 and 2015, relatively lower ‘total doses’ were estimated due to lower occupancy rates over local beaches. In 2016, a lower ‘total dose’ was due to both a reduction in the rate of mollusc consumption (from the revised habits data) and lower concentrations of plutonium radionuclides and americium-241 in molluscs. In 2017, the increase in ‘total dose’ was mostly attributed to a higher estimate of direct radiation from the site. Since 2018, the variation observed has been directly related to variation of gamma dose rates over substrates.
Figure 4.3. ‘Total dose’ at Heysham nuclear power stations, 2012 to 2023.
Year | Dose, mSv y-1 |
---|---|
Dose limit | 1 .0 |
2012 | 0.025 |
2013 | 0.028 |
2014 | 0.023 |
2015 | 0.023 |
2016 | 0.019 |
2017 | 0.025 |
2018 | 0.010 |
2019 | 0.018 |
2020 | 0.010 |
2021 | 0.015 |
2022 | 0.016 |
2023 | 0.015 |
Source specific assessments for high-rate terrestrial food consumption, and from external exposure for turf cutting over salt marsh, give exposures that were less than the ‘total dose’ in 2023 (Table 4.1). The estimated dose for terrestrial food consumption was 0.006mSv and up from less than 0.005mSv in 2022 (as amended in the RIFE 28 errata). The small increase in dose from terrestrial food consumption was mostly attributed to a higher maximum concentration of carbon-14 in milk measured in 2022. The estimated dose to the turf cutters was less than 0.005mSv in 2023 and unchanged from 2022. The dose to a local fisherman, who was considered to consume a large amount of seafood and was exposed to external radiation over intertidal areas, was 0.021mSv in 2023, which was approximately 2% of the dose limit for members of the public of 1mSv (Table 4.1). The dose to a local fisherman in 2022 was 0.022mSv. The main reason for the small decrease in dose was the same as that contributing to the maximum ‘total dose’.
Gaseous discharges and terrestrial monitoring
Both stations discharge gaseous radioactive waste via stacks to the atmosphere. Gaseous discharges from Heysham 1 and 2 in 2023 were broadly similar, in comparison with those in 2022. The monitoring programme for determining the effects of gaseous disposals was similar to that for other power stations. Data for 2023 are given in Table 4.3(a). The effects of gaseous disposals from the site were not easily detectable in foodstuffs in 2023. Unlike in 2022, sulphur-35 was positively detected in silage and beetroot in 2023. As in 2022, the carbon-14 concentrations in milk were above the default value used to represent background in 2023 but were higher than those observed in 2022. Tritium, gross alpha and gross beta concentrations in freshwater were below the investigation levels for drinking water in the Water Supply (Water Quality) (Amendment) 2018 Regulations (retained from the European Directive 2013/51).
Liquid waste discharges and aquatic monitoring
Permitted discharges of radioactive liquid effluent are made via outfalls into Morecambe Bay. Liquid discharges from Heysham 1 and 2, in 2023 were broadly similar in comparison with 2022.
The monitoring programme for the effects of liquid disposals included sampling of fish, shellfish, sediment, seawater and measurements of gamma dose rates. For completeness, the data considered in this section include all of those for Morecambe Bay. A substantial part of the programme is in place to monitor the effects of Sellafield disposals. The results for 2023 are given in Table 4.3(a) and Table 4.3(b). In general, activity concentrations in 2023 were similar (in comparison to those in recent years) and the effect of liquid disposals from Heysham was difficult to detect above the Sellafield background, based upon the greater historical discharges from Sellafield and monitoring data prior to operation of Heysham 1 and 2. Plutonium radionuclides and americium-241 concentrations in mussels and concentrations of technetium-99 in marine samples originating from Sellafield discharges were similar to those observed in 2022. As in recent years, strontium-90 was detected at low concentrations (reported as just above, or close to, the less than value) in food samples collected in 2023. Detectable concentrations of caesium-137 were mainly due to disposals from Sellafield and fallout from nuclear weapons testing (Figure 4.4). Gamma dose rates measured over sand were lower in 2023 (in comparison to 2022). Concentrations of caesium-137 in sediment samples near the Heysham nuclear power stations is given in Figure 4.4 over the period 2012 to 2023. There has been variability from year to year, with a large Sellafield influence.
Figure 4.4. Caesium-137 concentration in marine sediments near Heysham nuclear power stations between 2012 to 2023. Data are presented to 2 significant figures.
Year | 137Cs concentration Bq kg-1 (dry) |
---|---|
2012 | 25 |
2013 | 46 |
2014 | 33 |
2015 | 16 |
2016 | 36 |
2017 | 27 |
2018 | 24 |
2019 | 28 |
2020 | 23 |
2021 | 48 |
2022 | 40 |
2023 | 53 |
4.1.3 Hinkley Point, Somerset
The Hinkley Point Power Station sites are situated on the Somerset coast, west of the River Parrett estuary. There are 2 separate stations, A and B, that include twin Magnox reactors and twin AGRs, respectively. Hinkley Point A started electricity generation in 1965 and ceased in 2000. This station completed de-fuelling in 2004 and is undergoing decommissioning. Hinkley Point B ended power generation in August 2022 and has now entered the de-fuelling phase. In RIFE, a single environmental monitoring programme covers the effects of the 2 power stations, because the discharge effects from both sites impact on the same area. The most recent habits survey was conducted in 2017 (Greenhill and others, 2018).
The construction of the two new generation EPRTM reactors continues at Hinkley Point C. Summary details of earlier environmental permits issued (by the Environment Agency), the pre-construction safety case (approved by the ONR), the planning consents granted, and other approvals, are available in earlier RIFE reports (for example (Environment Agency, Food Standards Agency, Food Standards Scotland, Natural Resources Wales and others, 2019)) and from Hinkley Point decisions on environmental permit applications for a proposed new nuclear power station. Hinkley Point C is now expected to begin electricity generation in 2030. The latest information on Hinkley Point C can be found at: Hinkley Point on Gov.uk.
Doses to the public
In 2023, the ‘total dose’ from all pathways and sources of radiation was 0.032mSv (Table 4.1), or approximately 3% of the dose limit to members of the public. This is an increase from 0.015mSv in 2022. The representative person was an adult spending time over sediments, a change from 2022 (prenatal children of adults spending time over sediments). The increase in ‘total dose’ was mostly because higher gamma dose rates were measured over mud and rock at Stolford, from one year to the next.
The trend in ‘total dose’ over the period 2012 to 2023 is given in Figure 4.5. The low ‘total dose’ up until to 2016 was attributed to relatively lower gamma dose rates over local beaches. The increase in ‘total dose’ from 2017 to 2018 was mostly due to the increase in occupancy rates (over sand) reported in the most recent habits survey. The variation observed between 2019 and 2023 is mainly due to changes of gamma dose rates over mud substrates.
Figure 4.5. ‘Total dose’ at Hinkley Point nuclear power stations, 2012 to 2023.
Year | Dose, mSv y-1 |
---|---|
Dose limit | 1 .0 |
2012 | 0.013 |
2013 | 0.022 |
2014 | 0.022 |
2015 | 0.016 |
2016 | 0.013 |
2017 | 0.032 |
2018 | 0.041 |
2019 | 0.021 |
2020 | 0.023 |
2021 | 0.030 |
2022 | 0.015 |
2023 | 0.032 |
Source specific assessments for a high-rate consumer of locally grown food, and local people who consume high-rates of seafood and are exposed to external radiation over intertidal area, gave exposures that were less than the ‘total dose’ in 2023 (Table 4.1). The dose to this consumer of locally grown food was 0.006mSv in 2023 and unchanged from 2022 (0.006mSv as amended in the RIFE 28 errata).
The dose to the local fisherman was 0.020mSv in 2023, or 2% of the dose limit for members of the public of 1mSv. The reason for the increase in dose from 0.010mSv (in 2022) was the same as that contributing to the maximum ‘total dose’. This dose estimate also includes the effects of (historical) discharges of tritium from the former GE Healthcare Limited plant at Cardiff[footnote 16] and uses an increased dose coefficient (see Appendix 5). The estimated dose for a houseboat occupant was 0.027mSv in 2023 and up from 0.013mSv in 2022. The main reason for the increase was mostly due to higher gamma dose rates measured over different substrates at Blue Anchor Bay in comparison to 2022. This estimate is determined as a cautious value (due to direct measurements beneath houseboats not being available) and therefore not included in the ‘total dose’ assessment.
Gaseous discharges and terrestrial monitoring
Gaseous radioactive waste is discharged via separate stacks to the local environment. Discharges of carbon-14, sulphur-35 and argon-41 (reported as nil) from Hinkley Point B decreased in 2023 in comparison with 2022, due to cessation of power generation. Analyses of milk, fruit, honey and crops were undertaken to measure activity concentrations of tritium, carbon-14, sulphur-35 and gamma-emitting radionuclides. Local reservoir water samples were also analysed. Data for 2023 are given in Table 4.4(a). Activity concentrations of tritium and gamma-emitting radionuclides (cobalt-60 and caesium-137) in all terrestrial materials were reported as less than values. Unlike in 2022, sulphur-35 was positively detected in food samples (blackberries and wheat) in 2023. The carbon-14 concentrations in locally produced milk were higher than values observed in 2022. Carbon-14 was also detected in blackberries (as in previous years) above the expected background value in 2023. Tritium, gross alpha and gross beta concentrations in reservoir water were below the investigation levels for drinking water in the Water Supply (Water Quality) (Amendment) 2018 Regulations (retained from the European Directive 2013/51).
Liquid waste discharges and aquatic monitoring
Permitted discharges of radioactive liquid effluent from both power stations are made via separate outfalls into the Bristol Channel. Discharges of tritium from Hinkley Point B decreased in 2023 in comparison with 2022, due to the cessation of power generation. Analyses of seafood and marine indicator materials and measurements of external radiation were conducted over intertidal areas. The environmental results for 2023 are given in Table 4.4(a) and Table 4.4(b). Overall, activity concentrations observed in seafood and other materials from the Bristol Channel were generally similar to those in previous years. As in 2022, tritium was positively detected in seafood (shrimps, grey mullet and European oyster) in 2023. Concentrations of other radionuclides in the aquatic environment represent the combined effect of releases from these stations, plus other establishments that discharge into the Bristol Channel. Other contributors to the aquatic environment are Sellafield, and fallout from Chernobyl and nuclear weapons testing. Due to the low concentrations detected, it is generally difficult to attribute the results to a particular source. The concentrations of transuranic nuclides in seafoods were of negligible radiological significance. There is continuing evidence to suggest that caesium-137 concentrations in sediment have been generally decreasing over the reported years (Figure 4.6). Overall, gamma dose rates over intertidal substrates in 2023 were generally higher (where comparisons can be made for similar ground types and locations), in comparison to those observed in 2022.
Figure 4.6. Caesium-137 concentration in marine sediments near Hinkley Point nuclear power stations between 2012 to 2023. Data are presented to 2 significant figures.
Year | 137Cs concentration Bq kg-1 (dry) |
---|---|
2012 | 14 |
2013 | 18 |
2014 | 15 |
2015 | 11 |
2016 | 10 |
2017 | 13 |
2018 | 12 |
2019 | 12 |
2020 | 20 |
2021 | 9.2 |
2022 | 7.6 |
2023 | 6.9 |
4.1.4 Hunterston, North Ayrshire
Hunterston Power Station is located on the Ayrshire coast near West Kilbride and consists of 2 separate nuclear power stations - Hunterston A and Hunterston B.
Hunterston A was powered by twin Magnox reactors until it ceased electricity production in 1990 and is now being decommissioned by Nuclear Restoration Services (NRS). De-fuelling was completed in 1995. Decommissioning activities continue to focus on 2 major areas: the ongoing decommissioning of the cartridge (nuclear fuel) cooling pond; and making progress towards ensuring that all higher activity waste is stored in a passively safe manner.
Most of the radioactivity in liquid effluent discharged from the Hunterston A site over the last few years has arisen from the cartridge cooling pond. The draining of the cartridge cooling pond is now largely complete. However, there is still a need to manage the remaining radioactive sludges from several areas associated with the pond.
In October 2023, SEPA received an application from NRS to vary the permit, made under the Environmental Authorisations (Scotland) Regulations 2018 (EASR18), for the Hunterston A site. The variation application includes the addition of the Solid Intermediate Level Waste Encapsulation plant (SILWE) facility discharge stack to the site permit, and an increase to the site gaseous discharge limits. The permit variation application is expected to go to public consultation during 2024.
In terms of safe management of legacy higher activity waste at Hunterston A, NRS are in the process of commissioning the SILWE. The Wet Intermediate Level Waste Retrieval and Encapsulation Plant (WILWREP) is in the process of finalising modifications in order to process radioactively contaminated acid wastes. Processing of the legacy higher activity waste, present at the Hunterston A site will be processed through either SILWE or WILWREP, with the current plans being to make safe by encapsulating it in a grout mixture. The encapsulated waste will then be transferred to the Intermediate Level Waste Store (ILWS) for storage.
Hunterston B was powered by a pair of AGRs, referenced as Reactors 3 and 4. It ceased electricity production in January 2022. The station is currently being defueled by EDF Energy Nuclear Generation Limited, and Reactor 3 is now defueled. Once it has achieved Fuel Free Verification, it will be transferred to the NDA Estate to be decommissioned by NRS. Both gaseous and liquid discharges are much lower during defueling than in the operational phase.
In terms of safe management of legacy higher activity waste at Hunterston B, the preferred option is to use the ILWS on Hunterston A. Optioneering exercises are being carried out on the optimal solution for the Operational Waste Processing Facility (OWPF), which will manage the higher activity wastes arising from the station’s operational life. The Decommissioning Waste Processing Facility (DWPF) will be refurbished and used to manage the lower activity waste arising from the decommissioning phase.
Environmental monitoring in the area considers the effects of both Hunterston A and Hunterston B sites together. The most recent habits survey was conducted in 2017 (Dale, Smith, Tyler, Copplestone D, Varley, Bradley, Bartie and others, 2021). A new habits survey is scheduled to be undertaken in 2024.
Doses to the public
The ‘total dose’ from all pathways and sources of radiation was 0.013mSv in 2023 or approximately 1% of the dose limit (Table 4.1) to members of the public. This is an increase from less than 0.005mSv in 2022. The representative person was an adult consuming game meat, a change from that in 2022 (adults living near the site). The increase in dose was mostly due to a higher concentration of caesium-137 in venison samples. The trend in ‘total dose’ over the period 2012 to 2023 is given in Figure 4.7, doses have generally decreased from its peak in 2012 to 2023, with some variability over this period.
Figure 4.7. ‘Total dose’ at Hunterston nuclear power stations, 2012 to 2023. (Small doses less than or equal to 0.005mSv are recorded as being 0.005mSv).
Year | Dose, mSv y-1 |
---|---|
Dose limit | 1 .0 |
2012 | 0.032 |
2013 | 0.021 |
2014 | 0.021 |
2015 | 0.025 |
2016 | 0.021 |
2017 | 0.023 |
2018 | 0.005 |
2019 | 0.005 |
2020 | 0.005 |
2021 | 0.006 |
2022 | 0.005 |
2023 | 0.013 |
The estimated dose for seafood consumption was less than 0.005mSv in 2023, and unchanged from 2022.
The estimated dose for a terrestrial food consumer was 0.006mSv in 2023, which was less than 1% of the dose limit for members of the public of 1mSv and slightly down in comparison to that in 2022 (0.007mSv).
Gaseous discharges and terrestrial monitoring
Gaseous discharges are made via separate discharge points from the Hunterston A and Hunterston B stations. Hunterston A is in the decommissioning phase, and thus gaseous discharges from the site are low. Hunterston B defueled Reactor 3 in September 2023 and has begun defueling Reactor 4. As a consequence of being shut down, the gaseous discharges of argon-41 and iodine-131 (reported as nil) and, to a lesser extent, carbon-14 decreased in 2023, in comparison with 2022.
There is a substantial terrestrial monitoring programme which includes the analyses of a comprehensive range of wild and locally produced foods. In addition, air, freshwater, grass and soil are sampled to provide background information. The results of terrestrial food and air monitoring in 2023 are given in Table 4.5(a) and Table 4.5(c). The concentrations of radionuclides in air, milk, crops and fruit were generally low and similar to those in previous years (where comparisons can be made). The concentrations of ceasium-137 in venison were higher in 2023 in comparison to those in 2022. As in 2022, sulphur-35 was positively detected in grass and europium-155 was positively detected in soil in 2023. Tritium, gross alpha and gross beta concentrations in freshwater were below the investigation levels for drinking water in the Water Supply (Water Quality) (Amendment) 2018 Regulations (retained from the European Directive 2013/51).
Most of the activity concentrations in air at locations near to the site (Table 4.5(c)) were reported as less than values. Solid waste transfers in 2023 are also given in Appendix 1 (Table A1.4).
Liquid waste discharges and aquatic monitoring
Authorised liquid discharges from both Hunterston stations are made to the Firth of Clyde via the Hunterston B station’s cooling water outfall, with lower discharges of tritium and sulfur-35, due to the cessation of power generation. The primary source of liquid discharges from Hunterston A, is the cartridge cooling pond, draining of which is nearly complete (reducing the radiological hazards on site), which is supported by zero discharges of plutonium-241. The liquid discharges of tritium and, to a lesser extent, sulphur-35, from Hunterston B decreased in 2023, in comparison to those in 2022.
The main part of the aquatic monitoring programme consists of sampling of fish and shellfish and the measurement of gamma and beta dose rates on the foreshore. Samples of sediment, seawater and seaweed are analysed as environmental indicator materials.
The results of aquatic monitoring in 2023 are shown in Table 4.5(a) and Table 4.5(b). The concentrations of artificial radionuclides in the marine environment are predominantly due to Sellafield discharges, the general values being consistent with those to be expected at this distance from Sellafield. As in recent years, the concentrations of Sellafield derived technetium-99 in crabs and lobsters around Hunterston were low and all cobalt-60 concentrations in sediments were reported as less than value in 2023. In 2023, plutonium and americium-241 concentrations in seafood were generally similar to those observed in 2022. Gamma dose rates were generally similar in 2023, in comparison to those observed in recent years. Measurements of the beta dose rates over sand are reported as less than values in 2023. Caesium-137 concentrations in sediment have remained low over the last decade (Figure 4.8).
Figure 4.8. Caesium-137 concentration in marine sediments near Hunterston nuclear power stations between 2012 to 2023. Data are presented to 2 significant figures.
Year | 137Cs concentration Bq kg-1 (dry) |
---|---|
2012 | 4.0 |
2013 | 3.2 |
2014 | 4.7 |
2015 | 3.6 |
2016 | 5.6 |
2017 | 3.9 |
2018 | 3.9 |
2019 | 4.3 |
2020 | 3.8 |
2021 | 5.3 |
2022 | 4.1 |
2023 | 3.4 |
4.1.5 Sizewell, Suffolk
The two Sizewell Power Stations are located on the Suffolk coast, near Leiston. Sizewell A is a Magnox twin reactor site that ceased electricity generation in 2006. De-fuelling commenced in 2007 and was completed in 2014. Sizewell B, powered by one reactor, is the only commercial PWR power station in the UK. The Sizewell B power station began operation in 1995 and whilst the end of power generation is currently scheduled for 2035, the site operator is investigating extending operations to 2055 and potentially longer. The most recent habits survey was conducted in 2015 (Garrod, Clyne & Papworth 2016). In 2021, the permit for Sizewell B was varied to increase the annual permitted limit of carbon-14 to the atmosphere. The variation returned the limit from 500GBq to the original authorisation limit of 600GBq, due to changing practices. Further information on the variation, public consultation and our decision can be found at Sizewell B permit variation and in Appendix 1 (Table A1.1).
In 2020, Sizewell C Limited (previously NNB GenCo (SZC) Limited) applied to the ONR for a nuclear site license for Sizewell C, the Environment Agency for a radioactive substances activities permit and the Planning Inspectorate for a Development Consent Order (DCO) for Sizewell C where EDF Energy have proposed construction of 2 EPRTM reactors. Examination of the DCO application concluded in October 2021. In July 2022, the Secretary of State for the Department of Business, Energy and Industrial Strategy announced that the Sizewell C project had been granted its DCO. In March 2023, the Environment Agency granted the permit for discharging and disposing of radioactive waste in the environment. In May 2024, ONR granted its nuclear site license. The latest information can be found at: https://www.gov.uk/guidance/sizewell-nuclear-regulation.
Doses to the public
The ‘total dose’ from all pathways and sources of radiation was less than 0.010mSv in 2023 (Table 4.1) or approximately 1% of the dose limit to members of the public. This is a decrease from 0.011mSv in 2022 (as amended in the RIFE 28 errata). The representative person in 2023 was an adult living close to the site and a change from 2022 (an adult spending time over sediments). The decrease in ‘total dose’ was mostly attributed to lower estimate of direct radiation in 2023. The trend in ‘total dose’ over the period 2012 to 2023 is given in Figure 4.9. Any variation in ‘total dose’ from year to year was due to a change in the contribution from direct radiation from the site, that has been low in the recent years. The ‘total dose’ has declined (reduced by a factor of 5 or 6), following the closure of the Magnox reactors at Sizewell A in 2006 (Figure 4.1, (Environment Agency, Food Standards Agency, Food Standards Scotland, Natural Resources Wales and others, 2018)).
Figure 4.9. ‘Total dose’ at Sizewell nuclear power stations, 2012 to 2023.
Year | Dose, mSv y-1 |
---|---|
Dose limit | 1 .0 |
2012 | 0.021 |
2013 | 0.021 |
2014 | 0.020 |
2015 | 0.021 |
2016 | 0.021 |
2017 | 0.021 |
2018 | 0.026 |
2019 | 0.010 |
2020 | 0.017 |
2021 | 0.016 |
2022 | 0.011 |
2023 | 0.010 |
As in recent years, source specific assessments for both a high-rate consumer of locally grown foodstuffs, and of fish and shellfish, and of external exposure for houseboat occupancy, gave exposures that were also less than 0.005mSv in 2023 (Table 4.1).
Gaseous discharges and terrestrial monitoring
Gaseous wastes are discharged via separate stacks to the local environment. The discharges of carbon-14 decreased from Sizewell B in 2023, in comparison to those in 2022. This is a result of having a reactor outage in 2022, but not in 2023. The decrease in carbon-14 is also attributable to a change in behaviour of carbon delay-beds.
The results of the terrestrial monitoring in 2023 are shown in Table 4.6(a). As in recent years, gamma-ray spectrometry and radiochemical analysis of tritium and sulphur-35 in milk and crops generally showed very low concentrations of artificial radionuclides near the power stations in 2023. In 2023, carbon-14 concentrations in produced milk were just above the default value used to represent background level. Sulphur-35 and caesium-137 were positively detected at a very low concentration in a grass sample. As in 2022, tritium concentrations in local freshwater were reported as less than values in 2023. Tritium, gross alpha and gross beta concentrations in surface water were below the investigation levels for drinking water in the Water Supply (Water Quality) (Amendment) 2018 Regulations (retained from the European Directive 2013/51).
Liquid waste discharges and aquatic monitoring
Permitted discharges of radioactive liquid effluent are made via outfalls to the North Sea. The project to drain the fuel storage pond at Sizewell A was completed in 2019. This has resulted in a significant reduction in liquid effluent generation at Sizewell A. Small volumes of effluent were discharged by the foul sewer route via the sewage treatment works that is shared with Sizewell B. This route is monitored at quarterly intervals for tritium and caesium-137 for reassurance purposes. In the aquatic programme, analysis of seafood, seaweed, sediment, and seawater, and measurements of gamma dose rates were conducted in intertidal areas. Data for 2023 are given in Table 4.6(a) and Table 4.6(b). Concentrations of artificial radionuclides were low and mainly due to the distant effects of Sellafield discharges and fallout from Chernobyl and nuclear weapons testing. Unlike in 2022, tritium was positively detected in seafood (crab). As in 2022, concentrations of strontium-90 observed in sediment samples were all reported as less than values. Caesium-137 concentrations in sediment have remained low over the last decade and are generally decreasing over time (Figure 4.10). Overall, gamma radiation dose rates over intertidal areas were difficult to distinguish from the natural background and were similar to those reported in recent years.
Figure 4.10. Caesium-137 concentration in marine sediments near Sizewell nuclear power stations between 2012 to 2023. Data are presented to 2 significant figures.
Year | 137Cs concentration Bq kg-1 (dry) |
---|---|
2012 | 8.6 |
2013 | 7.0 |
2014 | 5.7 |
2015 | 6.7 |
2016 | 5.8 |
2017 | 5.8 |
2018 | 5.5 |
2019 | 4.6 |
2020 | 4.8 |
2021 | 4.3 |
2022 | 4.1 |
2023 | 4.4 |
4.1.6 Torness, East Lothian
Torness Power Station is located near Dunbar on the east coast of Scotland. The station is powered by twin AGRs and began operation at the end of 1987. The expected end of generation date for Torness remains as 2028. EDF keeps operational dates under constant review which has allowed them to provide the additional clarity on lifetime expectations.
The reactors continue to be offloaded and depressurised for refuelling. This has had an impact on the profile of discharges notably carbon-14, sulphur-35 and tritium. However, there has been no significant impact to the magnitude of the annual discharges.
The gaseous and liquid discharges from the site are given in Appendix 1.
The most recent habits survey, to determine the consumption and occupancy rates by members of the public, was undertaken in 2023 and publication is expected late 2024 (Scottish Environment Protection Agency no date).
Doses to the public
In 2023, the ‘total dose’ from all pathways and sources of radiation was 0.007mSv (Table 4.1), or less than 1% of the dose limit to members of the public. This is a slight increase from 0.006mSv in 2022. In 2023, the representative person was a prenatal child of a local inhabitant consuming domestic fruits, a minor change from a change of habits (prenatal children of local inhabitants consuming wild fruits and nuts in 2022). The trend in ‘total dose’ over the period 2012 to 2023 is given in Figure 4.11. The decrease in ‘total dose’ in the earlier years reflected a downward trend in the reported direct radiation, thereafter ‘total doses’ have remained broadly similar, from year to year, and were low.
Figure 4.11. ‘Total dose’ at Torness nuclear power station, 2012 to 2023. (Small doses less than or equal to 0.005mSv are recorded as being 0.005mSv).
Year | Dose, mSv y-1 |
---|---|
Dose limit | 1 .0 |
2012 | 0.020 |
2013 | 0.020 |
2014 | 0.020 |
2015 | 0.020 |
2016 | 0.021 |
2017 | 0.021 |
2018 | 0.005 |
2019 | 0.005 |
2020 | 0.006 |
2021 | 0.005 |
2022 | 0.006 |
2023 | 0.007 |
The source specific assessment for a high-rate consumer (infant) of terrestrial food gave an exposure that was 0.006mSv in 2023 (Table 4.1) and unchanged from 2022. The estimated dose to a high consumer of local fish and shellfish was less than 0.005mSv in 2023, or less than 0.5% of the dose limit for members of the public of 1mSv, and unchanged from that in recent years.
Gaseous discharges and terrestrial monitoring
A variety of foods, including milk, crops, fruit, and game as well as grass, soil and freshwater samples, were measured for a range of radionuclides. Air sampling at 3 locations was undertaken to investigate the inhalation pathway. The results of terrestrial food and air monitoring in 2023 are given in Table 4.7(a) and Table 4.7(c). Activity concentrations in many terrestrial foods are reported as less than values (or close to the less than value). The carbon‑14 concentrations in locally produced milk were close to the default value used to represent background. Caesium-137 was positively detected in partridge and in soil, but at low concentrations. As in recent years, americium-241 concentrations in all terrestrial food and soil samples (measured by gamma–ray spectrometry) were reported as less than values in 2023. The tritium, gross alpha and gross beta concentrations in freshwater were well below the investigation levels for drinking water in the Water Supply (Water Quality) (Amendment) 2018 Regulations (retained from the European Directive 2013/51). Measured concentrations of radioactivity in air, at locations near to the site, were all reported as less than values or close to the less than values in 2023 (Table 4.7(c)). Solid waste transfers in 2023 are also given in Appendix 1 (Table A1.4).
Liquid waste discharges and aquatic monitoring
Discharges of authorised liquid radioactive wastes are made to the Firth of Forth. Seafood, seaweed, sediment, and seawater samples were collected in 2023. Measurements were also made of gamma dose rates over intertidal areas, supported by analyses of sediment, and a beta dose rate on fishing gear. The results of the aquatic monitoring in 2023 are shown in Table 4.7(a) and Table 4.7(b). Concentrations of artificial radionuclides were mainly due to the distant effects of Sellafield discharges, and fallout from Chernobyl and nuclear weapons testing. Cobalt-60 was only detected positively in winkles in 2023. This activation product was likely to have originated from the station. Technetium-99 concentrations in marine samples were similar to those in recent years. Overall, caesium-137 concentrations in sediments have remained low over the last decade (Figure 4.12). Gamma dose rates over intertidal areas were generally indistinguishable from natural background and were similar to those measured in recent years. A measurement of the contact beta dose rate on fishermen’s pots was reported as less than values in 2023.
Figure 4.12. Caesium-137 concentration in marine sediments near Torness nuclear power station between 2012 to 2023. Data are presented to 2 significant figures.
Year | 137Cs concentration Bq kg-1 (dry) |
---|---|
2012 | 1.3 |
2013 | 1.4 |
2014 | 1.2 |
2015 | 3.6 |
2016 | 0.87 |
2017 | 3.0 |
2018 | 1.0 |
2019 | 0.96 |
2020 | 0.93 |
2021 | 0.82 |
2022 | 0.80 |
2023 | 0.59 |
4.2 Decommissioning sites
4.2.1 Berkeley, Gloucestershire and Oldbury, South Gloucestershire
Berkeley and Oldbury are both Magnox power stations. Berkeley Power Station is situated on the eastern bank of the River Severn and was powered by twin Magnox reactors. Berkeley was the first commercial power station in the UK to enter into decommissioning. Electricity generation started in 1962 and ceased in 1989. De-fuelling was completed in 1992. Decommissioning is still in progress and small amounts of radioactive wastes are still generated by these operations. Recently, a modular encapsulation plant went into service to treat intermediate level waste (ILW) to make it suitable for longer term storage and eventual disposal. The annual permitted limit of tritium to the atmosphere was increased to 1TBq in 2021 to allow treatment of ILW. Further details can be found in Appendix 1 (Table A1.1).
Oldbury Power Station is located on the south bank of the River Severn close to the village of Oldbury-on-Severn and has 2 Magnox reactors. Electricity generation started in 1967 and ceased in 2012. De-fuelling was completed in 2016, and the site is now prioritising the retrieval, treatment, and storage of intermediate level waste.
Berkeley and Oldbury sites are considered together for the purposes of environmental monitoring because the discharge effects from both sites impact on the same area. The most recent habits survey was undertaken in 2014 (Clyne, Garrod & Papworth 2015).
Doses to the public
In 2023, the ‘total dose’ from all pathways and sources of radiation was less than 0.005mSv (Table 4.1), or less than 0.5% of the dose limit to members of the public. This is unchanged from 2022 (as amended in the RIFE 28 Errata). The representative person was an adult spending time over sediments, a change from 2022 (infant milk consumers). The trend in the ‘total dose’ over the period 2012 to 2023 is given in Figure 4.13. Any longer-term variations in ‘total doses’ over time are attributable to changes in the contribution from direct radiation.
Figure 4.13. ‘Total dose’ at Berkeley and Oldbury nuclear power stations, 2012 to 2023. (Small doses less than or equal to 0.005mSv are recorded as being 0.005mSv).
Year | Dose, mSv y-1 |
---|---|
Dose limit | 1 .0 |
2012 | 0.014 |
2013 | 0.010 |
2014 | 0.005 |
2015 | 0.005 |
2016 | 0.006 |
2017 | 0.005 |
2018 | 0.005 |
2019 | 0.005 |
2020 | 0.005 |
2021 | 0.013 |
2022 | 0.005 |
2023 | 0.005 |
As in 2022, the source specific assessments for a high-rate consumer of fish and shellfish, in the vicinity of the Berkeley and Oldbury sites, gave exposures that was less than 0.005mSv in 2023 (Table 4.1). The dose to a consumer of fish and shellfish includes external gamma radiation and a component due to the tritium historically discharged from the former GE Healthcare Limited plant at Cardiff[footnote 16]. The estimated dose for a high-rate consumer (infant) of locally grown foods gave an exposure of less than 0.005mSv and was down from 0.005mSv in 2022. The decrease in dose was mostly due to lower concentrations of carbon-14 in milk, in comparison to those observed in 2022. The estimated dose for houseboat dwellers was 0.041mSv in 2023. This an increase from 0.009mSv in 2022. The reason for the increase in estimated dose for houseboat dwellers was due to the gamma dose rate recorded at Sharpness over salt march was higher in 2023, in comparison to the average dose rate over different substrates observed in 2022. The estimate for this pathway is determined as a cautious value (and therefore not included in the ‘total dose’ assessment), because gamma dose rate measurements used were not necessarily representative of the categories of ground type and houseboat location (as identified in the habits survey (Clyne and others, 2015)).
Gaseous discharges and terrestrial monitoring
The Berkeley and Oldbury sites discharge gaseous radioactive wastes via separate stacks to the atmosphere. Gaseous discharges of carbon-14 from Berkeley increased in 2023 in comparison with 2022, likely due to waste retrieval works on site. The focus of the terrestrial sampling was for the analyses of tritium, carbon-14 and sulphur-35 in milk and crops. Local freshwater samples were also analysed. Data for 2023 are given in Table 4.8(a). Unlike in 2022, sulphur-35 was detected positively in terrestrial food in 2023 (barley). Carbon-14 concentrations in milk were higher than those reported in recent years and above the default value used to represent background. Tritium, gross alpha and gross beta concentrations in surface water were below the investigation levels for drinking water in the Water Supply (Water Quality) (Amendment) 2018 Regulations (retained from the European Directive 2013/51).
Liquid waste discharges and aquatic monitoring
Liquid radioactive wastes are discharged to the Severn Estuary. Oldbury has ceased generation and was verified by the ONR as fuel free in 2016.
Analyses of seafood and marine indicator materials as well as measurements of external radiation were conducted over muddy intertidal areas. Data for 2023 are given in Table 4.8(a) and Table 4.8(b). Most of the artificial radioactivity detected was due to caesium-137, representing the combined effect of discharges from the sites, other nuclear establishments discharging into the Bristol Channel and fallout from nuclear weapons testing, and possibly a small Sellafield-derived component. Caesium-137 concentrations in sediment have been generally decreasing over the period between 2012 to 2023 (Figure 4.14). Unlike in 2022, technetium-99 was not positively detected in seaweed. Very small concentrations of other radionuclides were detected but taken together, were of low radiological significance. Gamma dose rates were generally higher than those observed in 2022.
Figure 4.14. Caesium-137 concentration in marine sediments near Berkeley and Oldbury nuclear power stations between 2012 to 2023. Data are presented to 2 significant figures.
Year | 137Cs concentration Bq kg-1 (dry) |
---|---|
2012 | 24 |
2013 | 20 |
2014 | 19 |
2015 | 22 |
2016 | 18 |
2017 | 16 |
2018 | 13 |
2019 | 16 |
2020 | 13 |
2021 | 12 |
2022 | 12 |
2023 | 11 |
4.2.2 Bradwell, Essex
The Bradwell site is located on the south side of the Blackwater Estuary. This Magnox power station ceased electricity production in 2002 after 40 years of operation, and de-fuelling was completed in 2006. In 2018, Bradwell became the UK’s first Magnox site to reach the interim end-stage of passive Care and Maintenance, following an accelerated decommissioning programme.
At the adjacent Bradwell B site, the Bradwell B Power Generation Company Limited (BrB) is in the early stages of developing its proposals for a new nuclear power station. In February 2022, the Environment Agency confirmed the HPR-1000 design (proposed for this site) was suitable for use in the United Kingdom.
Following the cessation of intermediate level waste (fuel element debris) treatment at Bradwell, the enhanced environmental monitoring reverted to the baseline monitoring programme in 2018. The results of the enhanced monitoring programme (2015 to 2017) are described in earlier RIFE reports (for example (Environment Agency, Food Standards Agency, Food Standards Scotland, Natural Resources Wales and others, 2018)).
The most recent habits survey was undertaken in 2015 (Clyne, Garrod & Ly 2016).
Doses to the public
The ‘total dose’ from all pathways and sources of radiation was less than 0.005mSv 2023 (Table 4.1), or less than 0.5% of the dose limit for members of the public of 1mSv. This is unchanged from 2022. The representative person in 2023 was an adult living on a houseboat, unchanged from 2022. The trend in ‘total dose’ over the period 2012 to 2023 is given in Figure 4.15. Any significant variations in ‘total dose’ over time were attributed to changes in the estimate of direct radiation.
Figure 4.15. ‘Total dose’ at Bradwell nuclear power station, 2012 to 2023. (Small doses less than or equal to 0.005mSv are recorded as being 0.005mSv).
Year | Dose, mSv y-1 |
---|---|
Dose limit | 1 .0 |
2012 | 0.005 |
2013 | 0.005 |
2014 | 0.005 |
2015 | 0.017 |
2016 | 0.036 |
2017 | 0.011 |
2018 | 0.011 |
2019 | 0.005 |
2020 | 0.005 |
2021 | 0.006 |
2022 | 0.005 |
2023 | 0.005 |
As in recent years, the doses to a high-rate consumer of fish and shellfish and a high-rate consumer of locally grown foods from source specific assessment were less than 0.005mSv in 2023.
Gaseous discharges and terrestrial monitoring
The power station is permitted to discharge gaseous wastes to the local environment via stacks to the atmosphere. Terrestrial sampling is similar to that for other power stations including analyses of milk and crop samples. Samples of freshwater are also taken from a coastal ditch. Data for 2023 are given in Table 4.9(a). Activity concentrations were low in terrestrial samples. Unlike in 2022, carbon-14 was detected in locally produced milk at concentrations close to the expected background concentration and tritium was not positively detected in the grass sample (lucerne). As in 2022, strontium-90 was positively detected in the coastal ditch freshwater sample collected near the turbine hall in 2023, but also between the power station and the shore. The gross beta activities in water from the coastal ditch were similar to those reported in recent years and continued to be enhanced above background concentrations, and in excess of the investigation levels for drinking water in the Water Supply (Water Quality) (Amendment) 2018 Regulations (retained from the European Directive 2013/51). However, the water in the ditches is not known to be used as a source of drinking water.
Liquid waste discharges and aquatic monitoring
Liquid wastes are discharged into the River Blackwater estuary. The source of this effluent is rainwater which is discharged to the estuary via the main drains pit at Bradwell site. The main drains pit is sampled at quarterly intervals. The site is also permitted to discharge non-radioactive effluent from the turbine hall voids to the main drains pit, and from there to the estuary. However, no effluent from this source was discharged in 2023. Effluent was last discharged to the estuary via Bradwell site’s active effluent system in 2017. This route has since been decommissioned and was removed from the site’s permit when the permit was last varied in 2019.
Aquatic sampling was directed at the consumption of locally caught fish and shellfish and external exposure over intertidal sediments. Seaweeds were also analysed as an environmental indicator material. Data for 2023 are given in Table 4.9(a) and Table 4.9(b). Low concentrations of artificial radionuclides were detected in marine samples as a result of discharges from the station, discharges from Sellafield and fallout from nuclear weapons testing. Due to the low concentrations detected, it is generally difficult to attribute the results to a particular source. There has been an overall decline in caesium-137 concentrations in sediments over the last decade (Figure 4.16). The caesium-137 concentrations observed in sediment samples collected in 2023 were similar to those reported in 2022 and were amongst the lowest reported values in recent years. Gamma dose rates on beaches were difficult to distinguish from natural background and were generally similar to those observed in recent years.
Figure 4.16. Caesium-137 concentration in marine sediments near Bradwell nuclear power station between 2012 to 2023. Data are presented to 2 significant figures.
Year | 137Cs concentration Bq kg-1 (dry) |
---|---|
2012 | 9.5 |
2013 | 7.7 |
2014 | 6.1 |
2015 | 6.2 |
2016 | 7.0 |
2017 | 6.7 |
2018 | 4.0 |
2019 | 3.9 |
2020 | 4.0 |
2021 | 3.2 |
2022 | 3.4 |
2023 | 3.2 |
4.2.3 Chapelcross, Dumfries and Galloway
Chapelcross was Scotland’s first commercial nuclear power station. It has 4 Magnox reactors and is located near the town of Annan in Dumfries and Galloway. After 45 years of continuous operation, electricity generation ceased in 2004, and the station has since been undergoing decommissioning. De-fuelling of the reactors began in 2008 and was completed during 2013. The major hazards remaining on the site are being addressed during the decommissioning phase.
Habits surveys have been undertaken to investigate aquatic and terrestrial exposure pathways. The most recent habits survey for Chapelcross was conducted in 2022 (Scottish Environment Protection Agency no date). In 2017, a separate habits survey was also conducted to determine the consumption and occupancy rates by members of the public on the Dumfries and Galloway coast (Smith and others, 2021). Copies of the most recent habits survey are available from SEPA by emailing [email protected]. The results of this survey are used to determine the potential exposure pathways relating to permitted liquid discharges from the Sellafield nuclear licensed site in Cumbria (see Section 3.3.1).
In 2023, the site applied for a variation to their EASR18 permit. The application was for increasing the gaseous discharge limits for all radionuclides apart from tritium, to support ongoing decommissioning activities.
Doses to the public
The ‘total dose’ from all pathways and sources of radiation was 0.010mSv in 2023 (Table 4.1), or 1% of the dose limit to members of the public. This is an increase from 0.008mSv in 2022. As in recent years, the representative person was an infant consuming locally produced milk at high rates. The small increase in dose was mainly due to the inclusion of the concentrations of carbon-14 in milk samples from the calculation of the ‘total dose’. The trend in ‘total dose’ over the period 2012 to 2023 is given in Figure 4.17, doses are generally low, with small peaks in 2013 and 2017 (still less than 5% of the dose limit to members of the public).
Figure 4.17. ‘Total dose’ at Chapelcross nuclear power station, 2012 to 2023.
Year | Dose, mSv y-1 |
---|---|
Dose limit | 1 .0 |
2012 | 0.011 |
2013 | 0.024 |
2014 | 0.014 |
2015 | 0.022 |
2016 | 0.026 |
2017 | 0.035 |
2018 | 0.019 |
2019 | 0.007 |
2020 | 0.018 |
2021 | 0.018 |
2022 | 0.009 |
2023 | 0.010 |
Source specific assessments for a high-rate consumer of locally grown food, and for a seafood (salmon) and wildfowl consumer, gave exposures that were less than the ‘total dose’ in 2023 (Table 4.1). The dose for the terrestrial food consumer was estimated to be 0.009mSv in 2023, up from 0.007mSv in 2022. The increase in dose was mostly due to the same reason as for the ‘total dose’. The dose for the seafood and wildfowl consumer was less than 0.005mSv in 2023 and unchanged from 2022. Crustacean consumption was not identified in the recent habits survey.
Doses from the presence of artificial radionuclides in marine materials in the Chapelcross vicinity are mostly due to the effects of Sellafield discharges and are consistent with values expected at this distance from Sellafield.
Gaseous discharges and terrestrial monitoring
Gaseous radioactive waste is discharged via stacks to the local environment.
Terrestrial monitoring consisted of the analysis of a variety of foods, including milk, fruit, crops as well as grass, soil and freshwater samples, for a range of radionuclides. Air samples at 3 locations were also monitored to investigate the inhalation pathway. The results of terrestrial food and air monitoring in 2023 are given in Table 4.10(a) and Table 4.10(c). Carbon-14 concentrations in milk were higher than those observed in 2022. As in 2022, americium-241 concentrations in all terrestrial food, and grass samples were all reported as less than values.
As in 2022, tritium was positively detected in the freshwater samples collected at Gullielands Burn and Black Esk. However, tritium, gross alpha and gross beta concentrations in all freshwater samples were well below the investigation levels for drinking water in the Water Supply (Water Quality) (Amendment) 2018 Regulations (retained from the European Directive 2013/51). Activity concentrations in air samples at locations near to the site (Table 4.10(c)) were reported as less than values (or close to the less than value). Solid waste transfers in 2023 are also given in Appendix 1 (Table A1.4).
Liquid waste discharges and aquatic monitoring
Radioactive liquid effluents are discharged to the Solway Firth. Unlike in previous years, there were no liquid discharges reported in 2023, as the site is currently in the decommissioning phase, where aqueous wastes are not being generated. Samples of seawater and seaweed (‘Fucus vesiculosus’), as environmental indicators, were collected in addition to shrimps, mussels, fish, sediments and measurements of gamma dose rates. Data for 2023 are given in Table 4.10(a) and Table 4.10(b). Concentrations of artificial radionuclides in marine materials in the Chapelcross vicinity are mostly due to the effects of Sellafield discharges and are consistent with values expected at this distance from Sellafield. Concentrations of most radionuclides remained similar to those detected in recent years. As in recent years, low concentrations of europium-155 were positively detected (reported as just above the less than value) in sediment samples.
As in previous years, concentrations of caesium-137, plutonium radionuclides and americium-241 were enhanced in sediment samples taken close to the pipeline in 2023. The average concentration of caesium-137 in sediments analysed in 2023 was slightly higher than in 2022 and is known to be largely due to Sellafield discharges (Figure 4.18). In 2023, gamma dose rates over intertidal sediment were similar to those in 2022 (where comparisons can be made). As in recent years, measurements of the contact beta dose rate on fishing nets and sediment were reported as less than values in 2023.
Figure 4.18. Caesium-137 concentration in marine sediments near Chapelcross nuclear power station between 2012 to 2023. Data are presented to 2 significant figures.
Year | 137Cs concentration Bq kg-1 (dry) |
---|---|
2012 | 50 |
2013 | 59 |
2014 | 72 |
2015 | 72 |
2016 | 59 |
2017 | 55 |
2018 | 56 |
2019 | 58 |
2020 | 97 |
2021 | 70 |
2022 | 39 |
2023 | 61 |
Between 1992 and 2009, several particles were found at the end of the discharge outfall consisting of limescale originating from deposits within the pipeline. Magnox Limited continues to monitor this area frequently and no particles were found during 2023 (as for the previous years). The relining of the pipeline and grouting at strategic points, which was undertaken between 2009 and 2010, has reduced the potential for particles to be released.
4.2.4 Dungeness, Kent
The Dungeness power stations are located on the south Kent coast, between Folkestone and Rye. There are 2 separate A and B nuclear power stations on neighbouring sites: station A was powered by twin Magnox reactors and station B has twin AGRs. Discharges are made via separate and adjacent outfalls and stacks, but for the purposes of environmental monitoring these are considered together. Dungeness A ceased generating electricity in 2006. De-fuelling of both Magnox reactors was completed in 2012. The Dungeness A site is currently undergoing decommissioning. In 2021, EDF Energy Nuclear Generation Limited decided to move Dungeness B nuclear power station into the defueling phase with immediate effect, following over 2 years of outage to deal with a range of technical issues. The most recent habits survey was conducted in 2019 (Greenhill and others, 2020).
Doses to the public
In 2023, the ‘total dose’ from all pathways and sources of radiation was less than 0.005mSv (Table 4.1), which was less than 0.5% of the dose limit to members of the public of 1mSv. This is a decrease from 0.011mSv in 2022. The decrease in ‘total dose’ was mainly attributed to a lower estimate of direct radiation from the site, in comparison to that in 2022. As in recent years, this was almost entirely due to direct radiation from the site and the representative person was an adult living near the site in 2023. The trend in ‘total dose’ over the period 2012 to 2023 is given in Figure 4.19. ‘Total doses’ ranged between 0.011 and 0.037mSv over this period and were dominated by direct radiation. Over a longer time-series, this dose has declined more significantly from the peak value of 0.63mSv, following the shutdown of the Magnox reactors in 2006 (Figure 4.1, (Environment Agency, Food Standards Agency, Food Standards Scotland, Natural Resources Wales and others, 2018)).
Figure 4.19. ‘Total dose’ at Dungeness nuclear power stations, 2012 to 2023. (Small doses less than or equal to 0.005mSv are recorded as being 0.005mSv).
Year | Dose, mSv y-1 |
---|---|
Dose limit | 1 .0 |
2012 | 0.015 |
2013 | 0.021 |
2014 | 0.021 |
2015 | 0.014 |
2016 | 0.021 |
2017 | 0.021 |
2018 | 0.022 |
2019 | 0.037 |
2020 | 0.012 |
2021 | 0.012 |
2022 | 0.011 |
2023 | 0.005 |
As in recent years, source specific assessments for a high-rate consumer of locally grown foodstuffs and a local bait digger, who consumes large quantities of fish and shellfish and spends long periods of time in the location being assessed for external exposure, give exposures that were less than the ‘total dose’ in 2023 (Table 4.1). The dose to a high-rate consumer of locally grown foods was estimated to be less than 0.005mSv and unchanged from 2022. The dose to a local seafood consumer was estimated to be 0.005mSv in 2023, and down slightly from 0.006mSv in 2022. As in recent years, the estimation of dose for a houseboat occupant (from external exposure) was not required in 2023 (consistent with information identified in the latest habits survey).
Gaseous discharges and terrestrial monitoring
Gaseous wastes are discharged via separate stacks to the local environment. The focus of the terrestrial sampling was the analyses of tritium, carbon-14 and sulphur-35 in milk and crops. The results of monitoring for 2023 are given in Table 4.11(a). Activity concentrations in many terrestrial foods are reported as less than values (or close to the less than value). Unlike in 2022, sulphur-35 was positively detected in grass. Unlike in 2022, tritium was not positively detected in food (potato) in 2023. As in recent years, carbon-14 was also detected in locally produced milk at concentrations slightly above the default value used to represent background. Tritium, gross alpha and gross beta concentrations in freshwater were below the investigation levels for drinking water in the Water Supply (Water Quality) (Amendment) 2018 Regulations (retained from the European Directive 2013/51) in 2023.
Liquid waste discharges and aquatic monitoring
Permitted discharges of radioactive liquid effluent from both power stations are made via separate outfalls to the English Channel. Dungeness B entered the defueling phase in June 2021. The draining of fuel ponds at Dungeness A was completed in 2019 and this removed the main source of aqueous waste discharges on site. Marine monitoring included gamma dose rate measurements, and analysis of seafood, seaweed, sediments and seawater. The results of monitoring for 2023 are given in Table 4.11(a) and Table 4.11(b). The caesium-137 concentrations in seafood are attributable to discharges from the stations, fallout from nuclear weapons testing and a long-distance contribution from Sellafield and La Hague. Due to the low concentrations detected in foods and marine materials, it is generally difficult to attribute the results to a particular source. The low concentrations of transuranic nuclides in molluscs (scallop sample collected in 2023) were typical of values expected at sites remote from Sellafield. As in recent years, all tritium results in seafood were reported as less than values in 2023. Caesium-137 concentrations in sediment have remained low over the last decade (Figure 4.20). Caesium-137 was detected at a very low concentrations (reported as just above the less than value) in sediment from Rye Harbour in 2023. Gamma dose rates recorded over substrates selected in 2023 were generally slightly higher than those observed in 2022.
Figure 4.20. Caesium-137 concentration in marine sediments near Dungeness nuclear power stations between 2012 to 2023. Data are presented to 2 significant figures.
Year | 137Cs concentration Bq kg-1 (dry) |
---|---|
2012 | 0.62 |
2013 | 0.35 |
2014 | 0.40 |
2015 | 0.46 |
2016 | 0.31 |
2017 | 0.24 |
2018 | 0.31 |
2019 | 0.28 |
2020 | 0.35 |
2021 | 0.30 |
2022 | 0.27 |
2023 | 0.56 |
4.2.5 Trawsfynydd, Gwynedd
Trawsfynydd Power Station is located inland on the northern bank of a lake in Eryri (Snowdonia) National Park, North Wales and was powered by twin Magnox reactors. Trawsfynydd stopped generating electricity in 1991. De-fuelling of the reactors was completed in 1995, and the station is being decommissioned. As part of NDA’s site-specific approach to decommissioning, Trawsfynydd was selected as the lead location, where the reactors will be dismantled without a long period of care and maintenance (Nuclear Decommissioning Authority 2021). The most recent habits survey was undertaken in 2018 (Greenhill, Clyne & Moore 2019).
Doses to the public
The ‘total dose’ from all pathways and sources of radiation was 0.007mSv in 2023 (Table 4.1), which was less than 1% of the dose limit to members of the public. This is a decrease from 0.009mSv in 2022. The representative person in 2023 was an adult exposed to external radiation over lake sediments and was unchanged from 2022. The decrease in ‘total dose’ was mostly attributed to lower caesium-137 concentrations in sediment (and hence calculated gamma dose rate over sand and stones) in 2023. The trend in ‘total dose’ over the period 2012 to 2023 is given in Figure 4.21, with variations in recent years due to estimates of direct radiation.
Figure 4.21. ‘Total dose’ at Trawsfynydd nuclear power station, 2012 to 2023. (Small doses less than or equal to 0.005mSv are recorded as being 0.005mSv).
Year | Dose, mSv y-1 |
---|---|
Dose limit | 1 .0 |
2012 | 0.025 |
2013 | 0.017 |
2014 | 0.013 |
2015 | 0.014 |
2016 | 0.019 |
2017 | 0.024 |
2018 | 0.017 |
2019 | 0.005 |
2020 | 0.012 |
2021 | 0.010 |
2022 | 0.009 |
2023 | 0.007 |
The dose to an angler (who consumes large quantities of fish and spends long periods of time in the location being assessed) was 0.006mSv in 2023 (Table 4.1), which was less than 1% of the dose limit for members of the public of 1mSv and down from 2022 (0.008mSv). The main reason for the decrease in dose was the same as for the ‘total dose’. The activity concentrations observed in lake sediments are used to calculate an external dose rate due to the difficulty in establishing the increase in measured dose rates above natural background rates. The dose to infants (1-year-old) consuming terrestrial food was 0.034mSv, or approximately 3% of the dose limit. This was slightly lower than in 2022 (0.037mSv) and the main reason for the small decrease in dose was because of lower maximum concentrations of americium-241 in milk samples collected in 2023.
Gaseous discharges and terrestrial monitoring
The results of the terrestrial programme, for local food (including milk) and grass samples in 2023, are shown in Table 4.12(a). Results from surveys, providing activity concentrations in sheep samples, are available in earlier RIFE reports (for example (Environment Agency, Food Standards Agency, Natural Resources Wales and others, 2014)). Concentrations of activity in all terrestrial samples were low. Tritium concentrations in all milk samples were reported as less than values. Like in 2022, carbon-14 concentrations in milk were reported just above the background concentration for milk. Measured activities for caesium-137 were reported as less than values (or close to the less than value) in 2023. The most likely source of small amounts of caesium-137 is fallout from Chernobyl and nuclear weapons testing, though it is conceivable that a small contribution may be made by re-suspension of lake activity. In recognition of this potential mechanism, monitoring of transuranic radionuclides, which are also associated with liquid discharges from the site (Ministry of Agriculture FIsheries and Food 1993), was also conducted on a potato sample. In 2023, detected activities of transuranic radionuclides in potatoes were low and generally similar to observations in other areas of England and Wales, where activity was attributable to fallout from nuclear weapons testing. Therefore, there was no direct evidence of re-suspension of activity in sediment from the lake shore contributing to increased exposure from transuranic radionuclides in 2023.
Liquid waste discharges and aquatic monitoring
Discharges of liquid radioactive waste are made to a freshwater lake, making the power station unique in UK terms. The aquatic monitoring programme was directed at consumers of freshwater fish caught in the lake and external exposure over the lake shoreline; the important radionuclides are caesium-137 and, to a lesser extent, strontium-90. Freshwater and sediment samples were also analysed in 2023. Habits surveys have established that the species of fish regularly consumed are brown and rainbow trout. Most brown trout are indigenous to the lake, but rainbow trout are introduced from a hatchery. Due to the limited period that they spend in the lake, introduced fish generally exhibit lower caesium-137 concentrations than indigenous fish.
Data for 2023 are given in Table 4.12(a) and Table 4.12(b). The majority of activity concentrations in fish and sediments result from historical discharges, due to inefficient dispersion and binding to sediments (Ministry of Agriculture FIsheries and Food 1993). As in recent years, due to sample availability, only rainbow trout samples were collected in 2023. The most recent brown trout sample to be collected was in 2015 and the concentration of caesium-137 was the lowest reported value for fish indigenous to the lake (Environment Agency, Food Standards Agency and others, 2016). As in previous years, caesium-137 concentrations in water samples are reported as less than values in 2023. Concentrations in the water column are predominantly maintained by processes that release activity (such as remobilisation) from near surface sediments. Caesium-137 concentrations in lake sediments were lower than those in 2022 at all sampling locations. In 2023, the highest caesium-137 concentration was in a sediment sample collected near the main pipeline (130Bq kg-1) and was lower than in 2022 (200Bq kg-1 collected on the lake shore near a café). Americium-241 was positively detected in two sediment samples (1.9 and 2.2Bq kg-1 near pipeline and southeast of a footbridge, respectively). In previous years’ monitoring, it has been demonstrated that these concentrations increase with depth beneath the sediment surface. As in 2022, the concentrations of strontium-90 and plutonium-238 in all sediment samples collected in 2023 were all reported as less than values and were similar to those in recent years. Unlike in 2022, plutonium-239+240 was positively detected in one sediment sample (collected near the main pipeline). Strontium-90 and transuranic concentrations in fish continued to be very low in 2023 and it is the effects of caesium-137 that dominate the external radiation pathways.
In the lake itself, there remains clear evidence for the effects of liquid discharges from the power station (activity concentrations of caesium-137, and other radionuclides, in sediments). However, gamma dose rates measured on the shoreline (where anglers fish) were difficult to distinguish from background dose rates in 2023 and were generally lower (where comparison could be made) to those in 2022. The predominant radionuclide in the dose assessment was caesium-137. The time trends of concentrations of caesium-137 in sediments and discharges are shown in Figure 4.22. A substantial decline in concentrations was observed in the mid to late 1990s in line with reducing discharges. Since 2000, the discharges of caesium-137 have generally decreased, but with some variability. Concentrations have generally decreased over the period 2000 to 2023, with fluctuations due to environmental variability and short periods of increased discharges, particularly in 2012 and 2013.
Figure 4.22. Caesium-137 liquid discharge from Trawsfynydd and concentration in sediment in Trawsfynydd Lake, 1994 to 2023.
4.2.6 Wylfa, Isle of Anglesey
Wylfa Power Station is located on the north coast of Anglesey and has 2 Magnox reactors. It was the last and largest power station of its type to be built in the UK and commenced electricity generation in 1971 and ceased in 2015. De-fueling operations were completed in 2019 (Nuclear Decommissioning Authority 2020). This milestone marked the end of de-fueling operations at all the UK’s first-generation nuclear reactors. In May 2024, the UK government chose Wylfa as the preferred site for another potential large-scale nuclear power plant.
The most recent habits survey was undertaken in 2023 (Greenhill and others, 2024).
Doses to the public
The ‘total dose’ from all pathways and sources of radiation was less than 0.005 mSv in 2023 (Table 4.1), which was less than 0.5% of the dose limit to members of the public. This is a decrease from 0.014mSv in 2022. The decrease in dose was almost entirely due to a lower estimate of direct radiation in 2023, compared to 2023. The representative person was an adult spending time over sediments and a change from 2022 (adults eating marine plants and algae and spending extensive time over sediments). The trend in ‘total dose’ over the period 2012 to 2023 is given in Figure 4.23. ‘Total doses’ remained broadly similar, from year to year, and were generally very low.
Figure 4.23. ‘Total dose’ at Wylfa nuclear power station, 2012 to 2023. (Small doses less than or equal to 0.005mSv are recorded as being 0.005mSv).
Year | Dose, mSv y-1 |
---|---|
Dose limit | 1 .0 |
2012 | 0.006 |
2013 | 0.005 |
2014 | 0.007 |
2015 | 0.013 |
2016 | 0.008 |
2017 | 0.005 |
2018 | 0.006 |
2019 | 0.005 |
2020 | 0.006 |
2021 | 0.005 |
2022 | 0.014 |
2023 | 0.005 |
Source specific assessments for a high-rate consumer of locally grown foods, and for a high-rate consumer of fish and shellfish (including external radiation) gave exposure levels that were lower than the ‘total dose’ (Table 4.1). The dose to a high-rate consumer of fish and shellfish (including external radiation) was less than 0.005mSv in 2023. The main reason for the decrease in dose (from 0.008mSv in 2022) was because of lower gamma dose rates measured over shingle at Cemlyn Bay West in 2023. The dose to a high-rate consumer of locally grown foods was less than 0.005mSv in 2023 and unchanged from 2022.
Gaseous discharges and terrestrial monitoring
Gaseous discharges in 2023 were similar in comparison to those in 2022.
The focus of the terrestrial sampling was for the analyses of tritium, carbon-14 and sulphur-35 in milk and crops. Data for 2023 are given in Table 4.13(a). Unlike in 2022, sulphur-35 was not detected positively in milk and, carbon-14 concentrations measured in locally produced milk were just above background levels.
Liquid waste discharges and aquatic monitoring
The aquatic monitoring programme consists of sampling of fish and shellfish, and the measurement of gamma dose rates. Samples of sediment, seawater and seaweed are analysed as environmental indicator materials. The results of the programme in 2023 are given in Table 4.13(a) and Table 4.13(b). The data for artificial radionuclides related to the Irish Sea continue to reflect the distant effects of Sellafield discharges. The activity concentrations in 2023 were similar to those in previous years. The reported concentration of technetium-99 in seaweed in 2023 (due to the distant effects of discharges to sea from Sellafield) was similar to levels observed in recent years. Caesium-137 concentrations in sediment have remained low over the last decade (Figure 4.24). Overall, gamma dose rates in 2023 were generally similar to those measured in recent years.
Figure 4.24. Caesium-137 concentration in marine sediments near Wylfa nuclear power station between 2012 to 2023. Data are presented to 2 significant figures.
Year | 137Cs concentration Bq kg-1 (dry) |
---|---|
2012 | 3.5 |
2013 | 3.4 |
2014 | 3.4 |
2015 | 2.7 |
2016 | 2.6 |
2017 | 3 .0 |
2018 | 2.6 |
2019 | 2.6 |
2020 | 2.2 |
2021 | 2.1 |
2022 | 3.0 |
2023 | 2.7 |
5 Research and radiochemical production establishments
Highlights
- ‘total doses’ (research) for the representative person were approximately 5% of the annual dose limit in 2023 (for sites that were assessed)
- ‘total doses’ (radiochemical production) for the representative person were less than 9% of the annual dose limit in 2023
Dounreay, Highland
- ‘total dose’ for the representative person was 0.028mSv and increased in 2023
GE Healthcare Limited, Grove Centre, Amersham, Buckinghamshire
- ‘total dose’ for the representative person was 0.089mSv and increased in 2023
- gaseous discharges of radon-222 increased in 2023
Harwell, Oxfordshire
- ‘total dose’ for the representative person was less than 0.005mSv and unchanged in 2023
Winfrith, Dorset
- ‘total dose’ for the representative person was 0.054mSv and increased in 2023
This section considers the results of monitoring near research establishments (Dounreay, Harwell, Winfrith and 1 minor research site) and 1 site associated with the radiopharmaceutical industry (Grove Centre, Amersham). One minor research site is considered in this section, the fusion energy research site at Culham which is not a nuclear licensed site.
The NDA owns the licensed nuclear sites at Harwell and Winfrith in England, and Dounreay in Scotland. The Harwell and Winfrith sites, previously operated by RSRL, were re-licensed in 2015 into a single site licensed company and merged to be part of Magnox Limited, also a wholly owned subsidiary of the NDA. In 2022, Dounreay Site Restoration Limited (DSRL) was the site licensed company for Dounreay, responsible for the decommissioning and clean-up of the Dounreay site. In April 2023 Dounreay joined with Magnox Limited. In October 2023, Magnox Limited changed its brand and became Nuclear Restoration Services (NRS).
All the nuclear research sites have reactors that are at different stages of decommissioning. Discharges of radioactive waste are largely related to decommissioning and decontamination operations and the nuclear related research that is undertaken. Some of this work is carried out by tenants or contractors.
The site at Amersham is operated by GE Healthcare Limited, a company manufacturing radiochemical products for the healthcare and life science research markets. A permit issued by the Environment Agency is in effect at the Amersham site, authorising the discharge of radioactive wastes.
Regular monitoring of the environment was undertaken near all sites to assess the effects of discharges. Independent monitoring of the environment around the sites is conducted by the Environment Agency, FSA and SEPA.
In 2023, gaseous and liquid discharges were below regulated limits for each of the establishments (see Appendix 1, Table A1.1 and Table A1.2). Solid waste transfers in 2023 from nuclear establishments in Scotland (Dounreay) are also given in Appendix 1 (Table A1.4). Data tables and the detailed results of the dose assessments, in Open Document Spreadsheet (ODS) format, are downloadable from the main RIFE page.
5.1 Dounreay, Highland
The Dounreay site was opened in 1955 to develop research reactors. Three reactors were built on the site: the Prototype Fast Reactor, the Dounreay Fast Reactor, and the Dounreay Materials Test Reactor. All 3 reactors are now shut down and undergoing decommissioning.
From 2005, the NDA became responsible for the UK’s civil nuclear liabilities, which included those at Dounreay. Consequently, the 3 existing radioactive waste disposal authorisations were transferred to a new site licensed company (Dounreay Site Restoration Limited, DSRL), before DSRL took over the site management contract. DSRL ownership was transferred to the NDA on 1st of April 2021. On the 1st of April 2023, Dounreay joined with Magnox Limited and SEPA transferred all environmental permits falling under its remit from DSRL to Magnox Limited. On 2nd of April 2024 Magnox Limited changed its company name to Nuclear Restoration Services Limited.
In 2023, radioactive waste discharges from the Dounreay site were made by DSRL and Magnox Limited (now known as Nuclear Restoration Services Ltd) under an EASR18 radioactive substances authorisation granted by SEPA.
The quantities of both gaseous and liquid discharges were generally similar to those released in recent years (Appendix 1, Table A1.1 and Table A1.2). Solid waste transfers from Dounreay in 2023 are also given in Appendix 1 (Table A1.4).
The most recent habits survey was conducted in 2018 (Dale, Smith, Tyler, Copplestone D, Varley, Bradley & Bartie 2021). This habits survey did not identify any occupancy in the area of Oigin’s Geo (see Figure 5.2), as an external exposure pathway.
In 2013, SEPA granted an authorisation to DSRL for the Low-Level Radioactive Waste Disposal Facility (LLWF) that is located adjacent to the main Dounreay site. The first phase of the disposal site comprised the construction and operation of 2 concrete vaults that began accepting low level radioactive waste and demolition low level waste from the Dounreay site in 2015. The safety case and planning permission allow for 2 additional construction phases, each comprising 2 vaults. Further phases of construction will be dependent on the progress with the decommissioning of the Dounreay site.
There are no authorised routes for liquid or gaseous discharges from the Dounreay LLWF. The facility is designed to contain the radioactive waste over a long time, allowing radioactive decay to occur while the waste remains isolated from the environment.
Doses to the public
The ‘total dose’ from all pathways and sources of radiation was 0.028mSv in 2023 (Table 5.1), or less than 3% of the dose limit to members of the public. This is an increase from 0.010mSv in 2022. The representative person was an adult consuming game meat at high rates (a change from an adult living near the site in 2022). The increase in ‘total dose’ was mostly due to the inclusion of game meat in the terrestrial monitoring programme conducted in 2023 (absent in 2022).
The trend in the annual ‘total dose’ over the period 2012 to 2023 is given in Figure 5.1. The variation observed between 2012 and 2013 was due to a change in caesium-137 concentration in game meat (rabbit), but ‘total doses’ were low. Between 2013 to 2015, the change in annual ‘total dose’ was mostly due to the contribution of goats’ milk not being included in the assessment (which had been assessed prior to 2013), as milk samples have not been available in recent years. As in 2023, the significant contributor that increased the dose was the concentration of caesium-137 found in venison (game) in 2016, 2018 and 2021.
Figure 5.1. ‘Total dose’ at Dounreay, 2012 to 2023.
Year | Dose, mSv y-1 |
---|---|
Dose limit | 1.0 |
2012 | 0.017 |
2013 | 0.012 |
2014 | 0.012 |
2015 | 0.010 |
2016 | 0.058 |
2017 | 0.010 |
2018 | 0.035 |
2019 | 0.010 |
2020 | 0.009 |
2021 | 0.026 |
2022 | 0.010 |
2023 | 0.028 |
The annual dose to a consumer of terrestrial foodstuffs was 0.017mSv in 2023, or less than 2% of the dose limit for members of the public of 1mSv, and up from 0.010mSv in 2022. The reason for the increase in dose was the same as that contributing to the ‘total dose’. As in previous years, adults were identified as the most exposed age group. The annual dose to a consumer of fish and shellfish, including external exposure from occupancy over local beaches, was lower than the ‘total dose’ in 2023 (0.006mSv) and down from 0.011mSv in 2022. The reason for the decrease in dose was mainly due to lower gamma dose rates over sand at Sandside Bay in comparison to those in 2022. The dose (external pathways only) to members of the public visiting Oigin’s Geo, based on previously collected habits data (Papworth, Garrod & Clyne 2014) and 2019 monitoring data, was less than 0.005mSv. The sample species/type and locations are listed in the respective site tables.
Figure 5.2. Monitoring locations at Dounreay, 2023 (not including farms or air sampling locations).
Gaseous discharges and terrestrial monitoring
In 2023, DSRL and Magnox Limited were authorised by SEPA to discharge radioactive gaseous wastes to the local environment via stacks to the atmosphere. The gaseous discharges of krypton-85, related to the removal of breeder fuel in the Dounreay Fast Reactor, increased in 2023 compared to 2022 (when no removal operations took place) and remains consistent with 2020 and 2021. The discharges also include a minor contribution from the adjoining reactor site (Vulcan naval reactor test establishment (NRTE)), which is operated by the MOD‘s Submarine Delivery Agency. Monitoring conducted in 2023 included the sampling of air, freshwater, grass, soil and locally grown terrestrial foods including meat (no game) and vegetables as well as wild foods. As there are no dairy cattle herds in the Dounreay area, no milk samples were collected from cattle. As in recent years, goats’ milk samples were not sampled, as no milk sample was available in 2023.
The sampling locations for the terrestrial (and marine) monitoring programmes are shown in Figure 5.2 (Dounreay) and Figure 5.3 (north of Scotland). Figure 5.3 also provides time trends of radionuclide discharges (gaseous and liquid), which are generally decreasing over the period 2012 to 2023. The sample species/type and locations are listed in the respective site tables. The results for terrestrial samples and radioactivity in air are given in Table 5.2(a) and Table 5.2(c).
Figure 5.3. Monitoring locations, discharges of gaseous and liquid radioactive wastes and monitoring of the environment in the north of Scotland, 2023 (not including farms or air sampling locations). The rectangle around the Dounreay site is the area presented in Figure 5.2.
The concentrations of radionuclides at Dounreay were generally low and relatively similar to those observed in previous years. As in recent years, strontium-90 was positively detected in a few food samples and antimony-125 and iodine-129 concentrations were all reported as less than values in 2023. The concentrations of plutonium-239+240 and americium-241 in strawberries and caseium-137 in venison were found to be elevated. Activity concentrations in air samples at locations near to the site (Table 5.2(c)) were reported as less than values, apart from gross alpha from the locations near Shebster and Balmore (just above the LoD).
Caesium-137 is likely to be present in terrestrial samples from the Dounreay area due to fallout from weapons testing in the 1960s and from the Chernobyl reactor accident in 1986. Caesium-137 was positively detected in venison (34 Bq kg-1) but at a similar level observed in recent years (31Bq kg-1 in 2021). No honey sample was available in 2023. Earlier RIFE reports have provided results and interpretation of honey monitoring (for example (Environment Agency, Food Standards Agency, Food Standards Scotland, Natural Resources Wales and others, 2018)).
Liquid waste discharges and aquatic monitoring
Low level liquid waste is routed via a Low-Level Liquid Effluent Treatment Plant (LLLETP). The effluent is discharged to sea (Pentland Firth) via a pipeline terminating 600 metres offshore at a depth of about 24 metres. The discharges also include groundwater pumped from the Dounreay shaft, surface water runoff, leachate from the on-site low level solid waste disposal facility (which operated from 1958 to 2005), and a minor contribution from the adjoining reactor site (Vulcan NRTE).
Routine marine monitoring included sampling of seafood and the measurement of beta and gamma dose rates. Seafood samples from within the zone covered by a FEPA[footnote 17] Order are collected under consent granted in 1997 by the Scottish Office and revised in 2011 by the FSS (then FSA in Scotland).
Cod, crab, mussel and winkle samples were collected from areas along the Caithness coastline. Additionally, sediment, seawater and seaweed were sampled in 2023 as indicator materials. The results for marine samples, and gamma and beta dose rates, are given in Table 5.2(a) and Table 5.2(b). Activity concentrations were generally low in 2023 and similar to those in recent years. Technetium-99 concentrations in seaweed remained at the expected levels for this distance from Sellafield and were similar to those in recent years (Figure 3.11). Figure 5.3 also gives time trend information for technetium-99 concentrations (from Sellafield) in seaweed at Sandside Bay (location shown in Figure 5.2), and Burwick. Data indicate a general decline in concentrations over the period at both locations. Caesium-137 concentrations in sediment at Oigin’s Geo (from 2013 to 2019), Sandside Bay and Rennibister (both 2012 to 2023), have generally decreases with variability. Overall, gamma dose rates in 2023 were similar to those observed in 2022, apart from those measured over sediment and the winkle bed at Sandside Bay, presenting lower gamma dose rates in comparison to 2022. As in 2022, beta dose rate measurements were all reported as less than values (Table 5.2(b)) in 2023.
During 2023, DSRL and Magnox Limited continued monitoring of local public beaches, using vehicle mounted detectors, for radioactive fragments in compliance with the requirements of the authorisation granted by SEPA. In 2023, 5 fragments were recovered from Sandside Bay but none from the Dounreay foreshore. The caesium-137 activity measured in the fragments recovered from Sandside Bay ranged between 5 and 44kBq (similar to ranges observed in recent years).
There were no fragments detected on the Dounreay foreshore and 4 fragments recovered from Sandside Bay during 2023. The depth of recovery and activity was within the normal range. The Particles Retrieval Advisory Group (Dounreay) (PRAG(D)) are preparing a report, due for release at the end of 2024. Dounreay particle updates are posted on Radioactive particles in the environment around Dounreay - GOV.UK (Dounreay Particles) and from October 2023 onwards particle find updates can be found here (onshore monitoring of radioactive particles).
The previously conducted offshore survey work provided data on repopulation rates of particles (fragments) to areas of the seabed previously cleared of particles. This work has improved the understanding of particle movements in the marine environment. The Dounreay Particles Advisory Group (DPAG) completed its work following the production of its Fourth Report (Dounreay Particles Advisory Group 2008). Since the work of DPAG[footnote 18] was concluded, PRAG(D) has published reports in 2010 and 2011 (Particles Retrieval Advisory Group (Dounreay) 2010; Particles Retrieval Advisory Group (Dounreay) 2011). In 2016, PRAG(D) published a further report into the retrieval of offshore particles. This was produced following an extensive research and monitoring programme in 2012 (Particles Retrieval Advisory Group (Dounreay) 2016). The report considered the extent and effectiveness of the offshore recovery programme to reduce the numbers of particles. The report concluded that any noticeable change in the rate or radioactive content of the particles arriving on the nearest public beach (Sandside Bay) will take several years to assess and recommended that in the interim the monitoring of local beaches should continue.
In 2007, the FSA reviewed the Dounreay FEPA Order. A risk assessment, that was peer-reviewed by UKHSA, indicated that the food chain risk was very small (Food Standards Agency 2009). The FEPA Order was reviewed with regard to ongoing work to remove radioactive particles from the seabed and the food chain risk. In 2009, the FSA in Scotland (now FSS) announced that the FEPA Order would remain in place and be reviewed again upon completion of the (now completed) seabed remediation work. Following a recommendation in the 2016 PRAG(D) report FSS agreed that the FEPA Order would remain in place and be reviewed following re-evaluation of particle arrival rates.
5.2 Grove Centre, Amersham, Buckinghamshire
GE Healthcare Limited’s sole remaining nuclear licenced site is located at Amersham, in Buckinghamshire. It consists of a range of plants previously used for manufacturing diagnostic imaging products for use in medicine and research, which are now closed and being decommissioned.
The monitoring programme consists of analysis of fish, crops, water, sediments and environmental materials, and measurements of gamma dose rates. The monitoring locations are shown in Figure 5.4. The sample species/type and locations are listed in the respective site tables. The most recent habits survey was undertaken in 2016 (Clyne and others, 2017).
Figure 5.4. Monitoring locations at Thames sites, 2023 (not including farms).
Doses to the public
The ‘total dose’ from all pathways and sources of radiation was conservatively estimated to be 0.089mSv in 2023 (Table 5.1) or less than 9% of the dose limit to members of the public. This was a slight increase from 2022 (0.086mSv). As in 2022, the dominant contribution to ‘total dose’ was from direct radiation from stored radioactive waste. The previous (pre-2022) dominant contribution (to ‘total dose’) was from direct radiation associated with radiochemicals manufactured elsewhere and brought to the Grove Centre site for storage and subsequent distribution. The Grove Centre is no longer used as a distribution hub. The representative person was an adult living in the vicinity of the site in 2023 and unchanged from 2022. Exposure from direct radiation varies around the boundary of the Grove Centre and therefore the ‘total dose’ is determined as a cautious upper value. The trend in annual ‘total dose’ over the period 2012 to 2023 is given in Figure 5.5. Up until 2013, ‘total doses’ were dominated by direct radiation. The lower values from 2014 onwards are due to changes in working practices for distribution activities, with products spending less time in the dispatch yard – as well as the construction of a shield wall on the western side of a building that contains legacy radioactive wastes. All distribution activity ceased in 2019. Dose from the site is now dominated by radiation from the waste store. The ‘total dose’ is expected to be no more than the conservative estimated value of 0.089mSv. The trends in ‘total dose’ is given in Figure 5.5. The doses are dominated by direct radiation, estimates of which have decreased in recent years.
Figure 5.5. ‘Total dose’ at Amersham, 2012 to 2023.
Year | Dose, mSv y-1 |
---|---|
Dose limit | 1.0 |
2012 | 0.22 |
2013 | 0.22 |
2014 | 0.14 |
2015 | 0.14 |
2016 | 0.15 |
2017 | 0.15 |
2018 | 0.14 |
2019 | 0.14 |
2020 | 0.14 |
2021 | 0.083 |
2022 | 0.086 |
2023 | 0.089 |
As in 2022, source specific assessments for a high-rate consumer of locally grown foods, for an angler and for a worker at Maple Lodge Sewage Treatment Works (STW), which serves the sewers to which permitted discharges are made, gave exposures that were less than the ‘total dose’ in 2023 (Table 5.1). The dose for a high-rate consumer of locally grown foods was 0.013mSv in 2023 and up from 2022 (0.010mSv). This increase in dose was mostly due to a higher contribution from the gaseous discharges of radon-222 in 2023. It should be noted that the current assessment methodology uses a conservative dose factor based on this nuclide being in equilibrium with its decay products. As in recent years, the dose to a local angler was less than 0.005mSv in 2023.
The 2016 habits survey at Amersham did not directly identify any consumers of fish, shellfish or freshwater plants. As in previous surveys, however, there were reports of occasional coarse fish and signal crayfish consumption (but no actual consumption rates). To allow for this, a consumption rate of 1kg per year for fish and crayfish has been included in the dose assessment for an angler.
The Grove Centre discharges liquid waste to Maple Lodge STW, and the proximity to raw sewage and sludge experienced by sewage treatment workers is a likely exposure pathway (National Dose Assessment Working Group 2004). The dose received by one of these workers was modelled using the methods described in Appendix 3. The dose from a combination of external exposure to contaminated raw sewage and sludge, inadvertent ingestion and inhalation of re-suspended radionuclides was less than 0.005mSv in 2023 and unchanged from recent years.
Gaseous discharges and terrestrial monitoring
The Amersham facility is permitted to discharge gaseous radioactive wastes via stacks on the site. Gaseous discharges of radon-222 increased, in comparison with those in 2022. Increased radon emission in 2023 are related to the retrieval and repackaging of radium wastes, which have resulted in higher radon emissions. This retrieval work is expected to be completed in 2025. Once retrievals are complete, radon emissions are expected to decrease significantly. The results for the terrestrial monitoring for 2023 are given in Table 5.3(a) and Table 5.3(b). As in recent years, sulphur-35 was positively detected in food (barley) and nuclear fallout derived caesium-137 was detected in soil near the site in 2023.
Liquid waste discharges and aquatic monitoring
Radioactive liquid wastes are discharged to sewers serving the Maple Lodge STW; treated effluent subsequently enters the Grand Union Canal and the River Colne. The results of the aquatic monitoring programme for 2023 are given in Table 5.3(a). As in 2022, activity concentrations in freshwater were mostly reported as less than values in 2023. Samples of effluent and sludge from Maple Lodge STW were also collected in 2023. The concentration levels observed in these samples were similar to the latest results observed in 2022 and before the COVID-19 pandemic in 2019 (Environment Agency and others, 2020). Tritium, gross alpha and gross beta concentrations observed in freshwater were below the investigation levels for drinking water in the Water Supply (Water Quality) (Amendment) 2018 Regulations (retained from the European Directive 2013/51). Gamma dose rates over grass were generally indistinguishable from natural background in 2023 (Table 5.3(b)) and were generally similar to those measured in recent years.
5.3 Harwell, Oxfordshire
The Harwell site was established in 1946 as the UK’s first Atomic Energy Research Establishment and is situated approximately 5km southwest of the town of Didcot. Since 2015, the Harwell site has been operated by Magnox Limited (now Nuclear Sites Restoration Ltd) on behalf of the NDA. The Harwell nuclear licensed site forms part of Harwell Campus, a science, innovation and business campus. The nuclear licensed site originally accommodated 14 experimental reactors, most of which have been completely removed. The fuel has been removed from the remaining 3 reactors, which are now in ‘care and maintenance’. In 2022, the final remediation work was completed for the decommissioned Liquid Effluent Treatment Plant (LETP). Radiological sampling to support the Environmental Permit surrender and delicensing of this area is now complete. Once the area is released from radioactive substances regulation, this land will be handed back to the Harwell Science Park for future development.
Other decommissioning work at the Harwell site is well underway with a project to transfer nuclear materials away from the site expected to be completed by 2025. Other historic wastes are being retrieved from their existing storage locations and repackaged for longer-term storage and eventual disposal. Intermediate level waste from Harwell, Winfrith and Culham will be stored in a designated facility on the Harwell site during a quiescent period of care and maintenance. At the final site clearance stage, the two materials test reactors (MTRs), DIDO and PLUTO, are due to be demolished and the remaining radioactive waste transferred to the GDF for final disposal. The most recent habits survey was conducted in 2015 (Clyne, Garrod & Dewar 2016).
Doses to the public
The ‘total dose’ from all pathways and sources of radiation was less than 0.005mSv in 2023 (Table 5.1), which was less than 0.5% of the dose limit to members of the public. This is unchanged from 2022. The representative person was an adult spending time over sediments. This is a change from 2022 (prenatal children of adults spending time over sediments). The trend in annual ‘total dose’ over the period 2012 to 2023 is given in Figure 5.6. The ‘total doses’ remained broadly similar, from year to year (up to 2016), and were low. The increase in ‘total dose’ in 2017 (from 2016), and then decrease in 2018, was attributed to changes in the estimate of direct radiation from the site.
Figure 5.6. ‘Total dose’ at Harwell, 2012 to 2023. (Small doses less than or equal to 0.005mSv are recorded as being 0.005mSv).
Year | Dose, mSv y-1 |
---|---|
Dose limit | 1.0 |
2012 | 0.018 |
2013 | 0.010 |
2014 | 0.016 |
2015 | 0.017 |
2016 | 0.015 |
2017 | 0.046 |
2018 | 0.028 |
2019 | 0.010 |
2020 | 0.008 |
2021 | 0.005 |
2022 | 0.005 |
2023 | 0.005 |
As in 2022, source specific assessments for a high-rate consumer of terrestrial foods, and for an angler, gave exposures that were less than 0.005mSv in 2023 (Table 5.1).
Gaseous discharges and terrestrial monitoring
Gaseous wastes are discharged via stacks to the local environment. As in previous years, discharges of radioactive wastes continued at very low rates, with some reported as nil in 2023. The monitoring programme sampled milk, fruit and cereal. Sampling locations at Harwell and in other parts of the Thames catchment are shown in Figure 5.4. The results of the terrestrial monitoring programme in 2023 are shown in Table 5.4. As in recent years, tritium and caesium-137 concentrations in terrestrial samples were all reported as less than values in 2023.
Liquid waste discharges and aquatic monitoring
Permitted discharges from Harwell are discharged to sewers serving the Didcot STW; treated effluent subsequently enters the River Thames at Long Wittenham. Discharges to the River Thames at Sutton Courtenay ceased in 2013, thereafter the decommissioning of the treated waste effluent discharge point was completed, and the discharge pipeline was removed. Further information is available via: Decommissioning of the Harwell pipeline.
Discharges of surface water drainage from the Harwell site are made via the Lydebank Brook, north of the site, which is a permitted route. As in recent years, discharges from Lydebank Brook were very low. Figure 5.7 shows trends of discharges over time (2012 to 2023) for cobalt-60 and caesium-137. There was an overall reduction in the discharges over the whole period and very low discharges in most recent years.
Figure 5.7. Trends in liquid discharges of cobalt-60 (part A) and caesium-137 (part B) from Harwell, Oxfordshire 2012 to 2023. Data are presented to 2 significant figures.
Year | 60Co discharge, MBq y-1 |
---|---|
2012 | 0.96 |
2013 | 0.52 |
2014 | 1.6 |
2015 | 1.0 |
2016 | 0.039 |
2017 | 0.29 |
2018 | 0.23 |
2019 | 0.45 |
2020 | 0.15 |
2021 | 0.25 |
2022 | 0.22 |
2023 | 0.13 |
Year | 137Cs discharge, MBq y-1 |
---|---|
2012 | 35 |
2013 | 35 |
2014 | 35 |
2015 | 7.7 |
2016 | 0.11 |
2017 | 2.7 |
2018 | 3.6 |
2019 | 3.6 |
2020 | 0.24 |
2021 | 0.17 |
2022 | 0.13 |
2023 | 0.10 |
The aquatic monitoring programme is directed at consumers of fish and occupancy (sediment and freshwater samples) close to the liquid discharge point. Due to on-going access issues at Day’s Lock, the Environment Agency have replaced this sampling location with River Thames above Shillingford. As in recent years, concentrations of tritium, cobalt-60 and caesium-137 in freshwater were reported as less than values. The caesium-137 concentration in sediment continued to be enhanced above background levels in 2023 but has low radiological impact. As in recent years, plutonium-239+240 was positively detected in the sediment sample collected near Shillingford. Unlike in 2022, plutonium-239+240 was positively detected in the sediment collected near Sutton Courtenay in 2023.
5.4 Winfrith, Dorset
The Winfrith site is located near Winfrith Newburgh, Dorset. It was established in 1957 as an experimental reactor research and development site. Since 2015, the Winfrith site has been operated by Nuclear Restoration Services (formerly Magnox Limited) on behalf of the NDA. At various times, there have been 9 research and development reactors. The last operational reactor at Winfrith closed in 1995. Seven of the reactors have been decommissioned. The final two; steam generating heavy water and ‘Dragon’ (high temperature gas-cooled) reactors and supporting site facilities, are in the process of being decommissioned. It is the end state intention of Magnox to return most of the land to a brownfield heathland site with public access.
Magnox Winfrith is located adjacent to the Tradebe-Inutec site. Tradebe-Inutec is an independent nuclear operator (see section 7.3). Magnox Winfrith and Tradebe-Inutec undertake separate site environmental monitoring programmes as required by their respective environmental permits. However, in RIFE, Magnox Winfrith and Tradebe-Inutec are considered together for the purposes of environmental monitoring because, with the exclusion of their liquid discharges (which discharge to different geographic areas), their operational activities and gaseous discharges impact on the same local area.
The most recent habits survey (covering both the Magnox and Tradebe-Inutec sites) was conducted in 2019 (Moore, Clyne & Greenhill 2020).
Doses to the public
In 2023, the ‘total dose’ from all pathways and sources of radiation was 0.054mSv (Table 5.1), or less than 6% of the dose limit to members of the public. This is an increase from 0.028mSv in 2022. The increase in ‘total dose’ was mainly attributed to a higher estimate of direct radiation from the site, in comparison to that in 2022. As in recent years, the ’total dose’ was almost entirely attributed to the direct radiation from the Magnox Winfrith site in 2023 (Table 1.1). The representative person was an adult living near the site and unchanged from 2022. Trends in annual ‘total doses’ over time are shown in Figure 5.8. At Winfrith, ‘total doses’ remained broadly similar from year to year (up to 2014) and were generally very low. The variations observed in recent years are mainly due to changes in the estimates of direct radiation from the site. The increase in estimates of total dose at Magnox Winfrith when compared to the previous reporting period, is attributed to the extent of the decommissioning work undertaken at the site in the recent years, including removal, temporary storage, and transfer of legacy wastes for off-site disposal. The specific waste transfer activities that occurred during 2023, are time limited in nature, and form part of the routine operational work required to secure the safe decommissioning of the site. Estimates of ‘total dose’ will fluctuate with operational decommissioning work demands as the site proceeds through to the final stage of decommissioning and all radioactive waste management activities cease.
Figure 5.8. ‘Total dose’ at Winfrith, 2012 to 2023. (Small doses less than or equal to 0.005mSv are recorded as being 0.005mSv).
Year | Dose, mSv y-1 |
---|---|
Dose limit | 1.0 |
2012 | 0.005 |
2013 | 0.005 |
2014 | 0.005 |
2015 | 0.014 |
2016 | 0.019 |
2017 | 0.038 |
2018 | 0.027 |
2019 | 0.027 |
2020 | 0.014 |
2021 | 0.006 |
2022 | 0.028 |
2023 | 0.054 |
As in 2022, the dose to a high-rate consumer of locally grown food was less than 0.005mSv in 2023. The dose to a high-rate consumer of fish and shellfish was 0.010mSv and up from less than 0.005mSv in 2022. The increase in dose was mostly due to a higher limit of detection value for americium-241 in fish (thornback ray) used in the assessment. Gaseous discharges and terrestrial monitoring
At Magnox Winfrith, gaseous radioactive waste is discharged via various stacks to the local environment. As in previous years, discharges were very low in 2023.
The main focus of the terrestrial sampling was the analyses of tritium and carbon-14 in milk and crops. Local freshwater, grass and sediment samples were also analysed. Sampling locations at Winfrith are shown in Figure 5.9. The sample species/type and locations are listed in the respective site tables. Data for 2023 are given in Table 5.5(a). Results from terrestrial samples provide little indication of an effect due to gaseous discharges. As in recent years, the carbon-14 concentrations in locally produced milk were above values used to represent background concentrations. Low tritium concentrations were measured in surface water to the north of the site, similar to those in previous years. Tritium, gross alpha, and gross beta concentrations in freshwater were below the investigation levels for drinking water in the Water Supply (Water Quality) (Amendment) 2018 Regulations (retained from the European Directive 2013/51).
Figure 5.9. Monitoring locations at Winfrith, 2023 (not including farms).
Liquid waste discharges and aquatic monitoring
Liquid wastes, from the Magnox Winfrith site, are disposed via a pipeline to deep water in Weymouth Bay. As in previous years, discharges continued at very low rates in 2023 (all reported as <1% of the annual limit).
Figure 5.10 shows trends of liquid discharges over time (2012 to 2023) for tritium and alpha-emitting radionuclides. In recent years, alpha-emitting radionuclide discharges have decreased since the peak observed in 2013. In comparison, tritium discharges have varied more between years, with periodic peaks in releases, due to operations at Tradebe-Inutec, but have also generally declined since 2015 and ceased in 2019.
Figure 5.10. Trends in liquid discharges of tritium (part A) and alpha emitting radionuclides (part B) from Winfrith, Dorset 2012 to 2023. Data are presented to 2 significant figures.
Year | Tritium discharge, TBq y-1 |
---|---|
2012 | 27 |
2013 | 9.8 |
2014 | 1.0 |
2015 | 11 |
2016 | 7.1 |
2017 | 1.0 |
2018 | 1.9 |
2019 | 0.00063 |
2020 | 0.028 |
2021 | 0.0008 |
2022 | 0.00036 |
2023 | 0.00022 |
Year | Alpha discharge, MBq y-1 |
---|---|
2012 | 93 |
2013 | 980 |
2014 | 11 |
2015 | 4.3 |
2016 | 4.7 |
2017 | 7.6 |
2018 | 4 |
2019 | 1.0 |
2020 | 0.81 |
2021 | 0.76 |
2022 | 0.53 |
2023 | 0.50 |
Analyses of seafood and marine indicator materials and measurements of external radiation over muddy intertidal areas were conducted. These are primarily to determine the effects of liquid discharges from the Magnox Winfrith site and, to a lesser extent, historical (pre-2019) liquid discharges from Tradebe-Inutec. Data for 2023 are given in Table 5.5(a) and Table 5.5(b). Concentrations of radionuclides in the marine environment were low and similar to those in previous years. Unlike in 2022, caesium-137 was not positively detected in the fish sample collected from Weymouth Bay (thornback ray) and technetium-99 was not detected in the seaweed sample collected from Bognor Rocks. Gamma dose rates in 2023 were generally slightly higher than those measured in 2022 (where comparison could be made).
5.5 Culham, Oxfordshire
Culham Centre for Fusion Energy (CCFE), operated by the United Kingdom Atomic Energy Authority (UKAEA), is the UK’s national laboratory for fusion research. CCFE hosts and is responsible for the operation of two experimental fusion reactors: the Joint European Torus (JET) and the Mega Amp Spherical Tokamak (MAST) Upgrade. The JET operational research programme is due to cease at the end of 2023, followed by a period of decommissioning.
The Culham site also operates several other facilities to support fusion energy and related technologies and should host commercial fusion developers to become a fusion cluster hub in the future.
An annual ‘total dose’ is not determined at this site in this report because an integrated habits survey has not been undertaken. The source specific dose (including tritium and caesium-137), from using the River Thames directly as a source of drinking water, downstream of the discharge point at Culham, was estimated to be 0.006mSv in 2023 (Table 5.1) and up from less than 0.005mSv in 2022. The main reason for the increase in dose was the use of a higher limit of detection for tritium observed in the freshwater samples collected from the River Thames downstream of the discharge point in the calculation of the dose.
Monitoring of soil and grass around Culham and of sediment and water from the River Thames was undertaken in 2023. Locations and data are shown in Figure 5.4 and Table 5.6, respectively. Historically, the main effect of the site’s operation was the increased tritium concentrations found in grass collected near the site perimeter. As in recent years, concentrations of tritium in all samples were reported as less than values. Overall, no effects were detected due to site operation. The reported caesium-137 concentration in the downstream sediment (<26Bq kg-1) was estimated to be lower in 2023, in comparison to that in recent years (26 and 23Bq kg-1 in 2022 and 2021, respectively). Caesium-137 concentrations in the River Thames sediment are not attributable to Culham but were most likely due to past discharges from Harwell and fallout from Chernobyl and nuclear weapons testing.
6 Defence establishments
Highlights
- ‘total doses’ for the representative person were approximately 4% of the dose limit for all sites assessed
Aldermaston, Berkshire
- ‘total dose’ for the representative person was 0.011mSv and increased in 2023
- gaseous discharges of volatile beta decreased in 2023
Barrow, Cumbria
- ‘total dose’ for the representative person was 0.042mSv and increased in 2023
Derby, Derbyshire
- ‘total dose’ for the representative person was 0.006mSv and increased in 2023
Devonport, Devon
- ‘total dose’ for the representative person was less than 0.005mSv and unchanged in 2023
Faslane and Coulport, Argyll and Bute
- ‘total dose’ for the representative person was <0.005mSv and decreased in 2023
Rosyth, Fife
- ‘total dose’ for the representative person was 0.008mSv and increased in 2023
This section considers the results of monitoring, under the responsibility of the Environment Agency, FSA, FSS and SEPA, undertaken routinely near 9 defence-related establishments in the UK. In addition, the MOD makes arrangements for monitoring at other defence sites where radiological contamination may occur. The operator at the Atomic Weapons Establishment (AWE) in Berkshire carries out environmental monitoring to determine the effects from discharges at its sites, including low level gaseous and liquid discharges from Burghfield, Berkshire. Monitoring at nuclear submarine berths is also conducted by the MOD (for example (Defence Science and Technology Laboratory 2023)).
In 2023, gaseous and liquid discharges were below regulated limits for each of the defence establishments, see Appendix 1, Table A1.1 and Table A1.2. Solid waste transfers in 2023 from nuclear establishments in Scotland (Coulport, Faslane, Rosyth and Vulcan) are also given in Appendix 1 (Table A1.4). Data tables and the detailed results of the dose assessments, in Open Document Spreadsheet (ODS) format, are downloadable from the main RIFE page.
6.1 Aldermaston and Burghfield
AWE has two major sites located in Berkshire: AWE Aldermaston and AWE Burghfield. AWE at Aldermaston provides and maintains the fundamental components of the UK’s nuclear deterrent, Trident, including component manufacture and radioactive waste management activities. The site and facilities at Aldermaston remain in government ownership under a government owned contractor operator (GOCO) arrangement. The day-to-day operations and the maintenance of the UK’s nuclear stockpile are managed, on behalf of the MOD, by AWE plc (which is a non-departmental public body, wholly owned by the MOD). AWE at Burghfield is responsible for the complex final assembly and maintenance of warheads whilst in service, as well as their decommissioning. Gaseous and liquid discharges are regulated by the Environment Agency, permitting discharges of low concentrations of radioactive waste to the environment, as well as their decommissioning.
The most recent habits survey to determine the consumption and occupancy rates by members of the public in the vicinity of the site was undertaken in 2022 (Greenhill and others, 2023).
Doses to the public
In 2023, the ‘total dose’ from all pathways and sources of radiation was 0.011mSv (Table 6.1), or approximately 1% of the dose limit to members of the public. This is an increase from less than 0.005mSv in 2022. As in 2022, the representative person was an adult consuming game meat at high rates. The increase in ‘total dose’ was attributed to a higher estimate of direct radiation from the Aldermaston site.
As in 2022, source specific assessments for high-rate consumers of locally grown foods, for sewage workers and for anglers, gave exposures that were also less than 0.005mSv in 2023 (Table 6.1). Estimates of activity concentrations in fish have been based on shellfish samples from the aquatic monitoring programme for the dose determination. A low consumption rate of 1kg per year for fish has been included in the dose assessment for anglers.
Gaseous discharges and terrestrial monitoring
Gaseous radioactive waste is discharged via stacks from facilities at Aldermaston and Burghfield Sites. Gaseous discharges of volatile beta decreased in comparison to those in 2022. These discharges are related to a nuclear forensics testing campaign, which was limited in 2023. Samples of milk, terrestrial foodstuffs, grass and soil were taken from locations close to the sites (Figure 5.4) and the results of the terrestrial monitoring in 2023 are given in Table 6.2(a). In 2023, tritium concentrations and other radionuclides in foodstuffs, including milk, were very low or reported as less than values. Caesium-137 concentrations were positively detected in grass and soil samples and were generally similar in both 2022 and 2021, where comparisons can be made. As in 2022, caesium-137 concentrations in all food samples were reported as less than values in 2023. Concentrations of uranium isotopes in milk in 2023 were lower than those values observed in 2022. Natural background or fallout concentrations from global nuclear weapons testing would have made a significant contribution to the detected values.
Liquid waste discharges and aquatic monitoring
Discharges of radioactive liquid effluent are made under permit to the sewage treatment works at Silchester (Figure 5.4), and to the Aldermaston Stream from the Aldermaston Site. A time-series trend of generally decreasing tritium discharges from Aldermaston, over the period 2012 to 2023, is shown in Figure 6.1. Tritium discharges have declined more significantly, over a longer period in comparison to the last decade (Figure 5.1, (Environment Agency, Food Standards Agency, Food Standards Scotland, Northern Ireland Environment Agency and others, 2018)). The longer-term decline in discharges is due to the replacement of the original tritium facility. This replacement facility uses sophisticated abatement technology that has resulted in significantly less tritium discharged into the environment and the reduction of historical groundwater contamination by radioactive decay and dilution by natural processes. Discharges of radioactive liquid effluent from Burghfield are made to a sewage treatment works located on the Burghfield Site. Environmental monitoring of the River Thames, at Pangbourne and Mapledurham, has continued to assess the effect of historical discharges.
Figure 6.1. Trends in liquid discharges of tritium from Aldermaston, Berkshire 2012 to 2023 (including discharges to Silchester sewer and Aldermaston Stream). Data are presented to 2 significant figures.
Year | Tritium discharge, GBq y-1 |
---|---|
2012 | 0.70 |
2013 | 0.63 |
2014 | 0.67 |
2015 | 0.11 |
2016 | 0.45 |
2017 | 0.51 |
2018 | 0.38 |
2019 | 0.41 |
2020 | 0.30 |
2021 | 0.35 |
2022 | 0.26 |
2023 | 0.28 |
Activity concentrations for freshwater, fish, sediment samples, including gully pot sediments from road drains, and measurements of gamma dose rates, are given in Table 6.2(a) and Table 6.2(b). As in recent years, the Environment Agency continued their enhanced environmental monitoring of sediments and freshwater samples in 2023. The concentrations of artificial radioactivity detected in the Thames catchment were very low in 2022 and generally similar to those in recent years. Tritium concentrations in freshwater samples were all reported as less than values. As in 2022, iodine-131 was not positively detected in seafood samples in 2023. Activity concentrations of artificial radionuclides in shellfish were very low in 2023 and similar to those reported in recent years. Analyses of caesium-137 and uranium activity concentrations in River Kennet sediments were broadly consistent with those in recent years. As in 2022, caesium-137 concentrations in gully pot samples were reported as less than values, or just above the less than value, and tritium concentrations in freshwater samples were all reported as less than values. Gross alpha and beta activities in freshwater samples were below the investigation levels for drinking water in the Water Supply (Water Quality) (Amendment) 2018 Regulations (based upon the European Directive 2013/51). Gamma dose rates were below or close to natural background.
6.2 Barrow, Cumbria
At Barrow, BAE Systems Marine Limited builds, tests and commissions new nuclear-powered submarines. Gaseous discharges were reported as nil and liquid discharges of tritium, carbon-14 and cobalt-60 to sewer were all very low (<1% of the annual limit) in 2023. The most recent habits survey was undertaken in 2012 (Garrod CJ and others, 2013b).
The ‘total dose’ from all pathways and sources of radiation was 0.042mSv (Table 6.1) in 2023, or approximately 4% of the dose limit to members of the public. This is an increase from 0.030mSv in 2022. Virtually all of this dose was due to the effects of Sellafield discharges. As in recent years, the representative person was an adult living on a local houseboat. The increase in ‘total dose’ was mostly due to gamma dose rates being measured over different substrates at Askam Pier, from one year to the next. The trend in ‘total dose’ over the period 2012 to 2023 is given in Figure 6.2, ranging from 0.030 to 0.083mSv.
Figure 6.2. Trends in ‘total dose’ at Barrow, in mSv y-1, 2012 to 2023
Year | Dose, mSv y-1 |
---|---|
Dose limit | 1 .0 |
2012 | 0.057 |
2013 | 0.076 |
2014 | 0.055 |
2015 | 0.051 |
2016 | 0.082 |
2017 | 0.074 |
2018 | 0.046 |
2019 | 0.057 |
2020 | 0.061 |
2021 | 0.044 |
2022 | 0.030 |
2023 | 0.042 |
As in 2022, source specific assessments for a person living on a local houseboat gave exposures that were less than the ‘total dose’ in 2023 (Table 6.1). The assessment for a high-rate consumer of locally grown food (0.013mSv) was higher than in 2022 (<0.005mSv). The apparent increase in dose was attributed to the inclusion of americium-241 activity concentrations in seafood in the 2023 assessment. In line with the rules on use of the results for dose calculations, americium-241 was excluded because detectable activity was found in grass samples from the terrestrial environment in 2023. No assessment of seafood consumption was undertaken in 2022 because of the absence of relevant monitoring data. However, the dose from seafood consumption is less important than that from external exposure on a houseboat (Environment Agency, Food Standards Agency, Northern Ireland Environment Agency and others., 2014).
The FSA’s terrestrial monitoring is limited to vegetable and grass (or silage) sampling. The Environment Agency monitors gamma dose rates and analysis of sediment samples from local intertidal areas and is directed primarily at the far-field effects of Sellafield discharges. The results are given in Table 6.3(a) and Table 6.3(b). No effects of discharges from Barrow were apparent in the concentrations of radioactivity in vegetables and silage, with most results reported as less than values. In 2023, the reported gross beta concentration, due to the far-field effects of Sellafield discharges, in Walney Channel sediment was slightly higher in comparison to that in 2022. The gamma dose rates in intertidal areas near Barrow in 2023 are given in Table 6.3(b) and Table 3.9. As in previous years, gamma dose rates were enhanced above those expected due to natural background, and generally higher than those measured in 2022. Any enhancement above natural background is most likely due to the far-field effects of historical discharges from Sellafield.
6.3 Derby, Derbyshire
Rolls-Royce Submarines Limited (RRSL), formerly Rolls-Royce Marine Power Operations Limited, a subsidiary of Rolls-Royce plc, carries out design, development, testing and manufacture of nuclear-powered submarine fuel at its 2 adjacent sites in Derby at Raynesway. Small discharges of liquid effluent are made via the Megaloughton Lane STW to the River Derwent and very low concentrations of alpha activity are present in releases to the atmosphere. Other wastes are disposed of by transfer to other sites, for example, at a permitted landfill site or by incineration. The most recent habits survey was undertaken in 2021 (Greenhill, Clyne & Moore 2022).
Doses to the public
The ‘total dose’ from all pathways and sources of radiation was 0.006mSv in 2023 (Table 6.1), which is less than 1% of the dose limit to members of the public. This is an increase from less than 0.005mSv in 2022. The representative person was an infant living near the site in 2023 (a change from an infant consuming locally sourced water 2022). The increase in ‘total dose’ and change in representative person was attributed to a higher estimate of direct radiation from the site. Source specific assessments for consumption of fish, crustaceans and drinking river water at high-rates, and spent time on river-washed areas also gave exposures that were also less than 0.005mSv in 2023 (Table 6.1).
Results of the routine monitoring programme at Derby are given in Table 6.3(a). Concentrations of uranium in samples taken around the site in 2023 were generally similar to those in previous years. More detailed analysis in previous years has shown the activity as being consistent with natural sources. The gross alpha and beta activities in water samples from the River Derwent were less than the investigation levels for drinking water in the Water Supply (Water Quality) (Amendment) 2018 Regulations (based upon the European Directive 2013/51). Caesium-137 detected in sediments from local water courses was most likely from historic fallout from overseas sources, such as nuclear weapons testing.
Table 6.3(a) also includes analytical results for a water sample taken from Fritchley Brook, downstream of Hilts Quarry, near Crich in Derbyshire. RRSL formerly used the quarry for the controlled burial of solid low level radioactive waste. Concentrations of uranium isotopes detected in the sample in 2023 were broadly similar to those reported elsewhere in Derbyshire (Table 8.7).
6.4 Devonport, Devon
The Devonport Royal Dockyard consists of 2 parts and is operated by His Majesty’s Naval Base (HMNB) (owned and operated by the MOD) and Devonport Royal Dockyard Limited (owned by Babcock International Group plc). Devonport Royal Dockyard refits, refuels, repairs and maintains the Royal Navy’s nuclear-powered submarine fleet. The Environment Agency has granted a permit to discharge liquid radioactive waste to the Hamoaze, which is part of the Tamar Estuary, and to the local sewer, and gaseous waste to the atmosphere.
The most recent habits survey to determine the consumption and occupancy rates by members of the public was undertaken in 2017 (Moore and others, 2018). The routine monitoring programme in 2023 consisted of measurements of gamma dose rate and analysis of barley, vegetables, fish, shellfish and other environmental indicator materials (Table 6.3(a) and Table 6.3(b)).
Doses to the public
The ‘total dose’ from all pathways and sources of radiation was less than 0.005mSv in 2023 (Table 6.1), which was less than 0.5% of the dose limit, and unchanged from 2022. The representative person was an adult consuming locally collected marine plants at high rates, who also consumed fish (which largely determined the exposure) and spent time on intertidal areas. The trend in annual ‘total doses’ at Devonport remains less than 0.005mSv (Figure 6.1, (Environment Agency, Food Standards Agency, Food Standards Scotland, Northern Ireland Protection Agency and others, 2019)).
As in 2022, source specific assessments for a high-rate consumer of locally grown food (including doses from external and inhalation from gaseous discharges), for fish and shellfish, and for an occupant of a houseboat, gave exposures that were also less than 0.005mSv in 2023 (Table 6.1).
Gaseous discharges and terrestrial monitoring
Terrestrial samples (grass and potatoes) were analysed for a number of radionuclides. Activity concentrations in terrestrial samples were reported as less than values in 2023, apart from a positive tritium result observed in potatoes.
Liquid waste discharges and aquatic monitoring
Discharges of tritium, cobalt-60 and ‘other radionuclides’ to the Hamoaze (estuary) were generally similar in 2023, in comparison to those releases in 2022. The trends of tritium and cobalt-60 discharges over time (2012 to 2023) are given in Figure 6.3. The main contributor to the variations in tritium discharges over time has been the re-fitting of Vanguard class submarines. These submarines have a high tritium inventory as they do not routinely discharge primary circuit coolant until they undergo refuelling at Devonport. Cobalt-60 discharges have declined more significantly than tritium, since the early 2000s (Figure 5.2, (Environment Agency, Food Standards Agency, Food Standards Scotland, Northern Ireland Environment Agency and others, 2018)). The underlying reason for the overall decrease in cobalt-60 discharges over nearly 3 decades has been the improvement in submarine reactor design so that less cobalt-60 was produced during operation, and therefore less was released during submarine maintenance operations.
Figure 6.3. Trends in liquid discharges of tritium (part A) and cobalt-60 (part B) from Devonport, Devon 2012 to 2023. Data are presented to 2 significant figures.
Year | Tritium discharge, TBq y-1 |
---|---|
2012 | 0.21 |
2013 | 0.086 |
2014 | 0.022 |
2015 | 0.048 |
2016 | 0.018 |
2017 | 0.029 |
2018 | 0.012 |
2019 | 0.005 |
2020 | 0.0084 |
2021 | 0.011 |
2022 | 0.0024 |
2023 | 0.0029 |
Year | 60Co discharge, GBq y-1 |
---|---|
2012 | 0.07252 |
2013 | 0.0595 |
2014 | 0.02513 |
2015 | 0.03473 |
2016 | 0.03867 |
2017 | 0.02832 |
2018 | 0.01605 |
2019 | 0.0047 |
2020 | 0.00319 |
2021 | 0.00262 |
2022 | 0.00248 |
2023 | 0.001798 |
In marine samples, concentrations of tritium and cobalt-60 were reported as less than values in 2023. Low caesium-137 concentrations, likely to originate from other sources (such as nuclear weapons testing), were measured in sediment samples. Carbon-14 concentrations in seafood species were generally similar to those in previous years. The seaweed and sewage sludge samples contained concentrations of iodine-131 in 2023. These were most likely to have originated from the therapeutic use of this radionuclide in local hospitals. Gamma dose rates in the vicinity of Devonport in 2023, were similar to those in recent years, and reflect the local effects of enhanced background radiation from natural sources.
6.5 Faslane and Coulport, Argyll and Bute
The HMNB Clyde establishment consists of the naval base at Faslane and the armaments depot at Coulport. Babcock Marine, a subsidiary of Babcock International Group plc, operates HMNB Clyde, Faslane in partnership with the MOD. However, the MOD remains in control of the undertaking, through the Naval Base Commander, Clyde (NBC Clyde) in relation to radioactive waste disposal. MOD through NBC Clyde also remains in control of the undertaking at Coulport although many of the activities undertaken at Coulport have been outsourced to an industrial alliance comprising of AWE plc, Babcock and Lockheed Martin UK (known as ABL).
Discharges of liquid radioactive waste into the Gare Loch from Faslane and the discharge of gaseous radioactive waste in the form of tritium to the atmosphere from Coulport, are made under Letters of Agreement (LoA) between SEPA and the MOD. The construction of a new radioactive waste treatment facility at Faslane continued in 2023 and work to commission the facility was started. An application for a revised LoA that includes discharges from the facility was submitted to SEPA in 2019. SEPA consulted on the application in 2020 and has been progressing with the determination of the application, which is expected to reach completion in 2024.
In 2023, gaseous tritium discharges (from Coulport) and liquid discharges (from Faslane) were broadly similar, in comparison to those releases in recent years (see Appendix 1, Table A1.1 and Table A1.2, respectively).
The disposal of solid radioactive waste from each site is made under a separate LoA between SEPA and the MOD. Solid waste transfers in 2023 are given in Appendix 1 (Table A1.4).
The most recent habits survey to determine the consumption and occupancy rates by members of the public was undertaken in 2023 (Scottish Environment Protection Agency no date).
The ‘total dose’ from all pathways and sources of radiation was less than 0.005mSv in 2023 (Table 6.1), which was less than 0.5% of the dose limit to members of the public. This is a decrease from 2022 (0.007mSv). The representative person was an adult consuming fish at high rates and unchanged from 2022. The apparent decrease in dose was attributed to the exclusion of americium-241 activity concentrations in seafood in the 2023 assessment. In line with the rules on use of the results for dose calculations, americium-241 was excluded because no detectable activity was found in any samples from the aquatic environment in 2023. Activity concentrations in fish (not collected in recent years) were estimated using reported environmental fish data in 2023, sampled outside the aquatic habits survey area of this site, but within the Firth of Clyde. The assessment of this ‘total dose’ was therefore highly conservative, due to the assumption of fish data. In 2023, both source specific assessments for a high-rate consumer of shellfish and a consumer of locally grown food, which is based on limited data, gave exposures of less than 0.005mSv. The main reason for the decrease in dose (from 0.010mSv in 2022) to the consumer of shellfish was the same as for the ‘total dose’. The dose to a consumer of locally grown food remains unchanged from 2022.
The routine marine monitoring programme consisted of the analysis of shellfish, seawater, seaweed and sediment samples, and gamma dose rate measurements. Terrestrial monitoring included meat, domestic fruit, honey, water, grass and soil sampling. The results in 2023 are given in Table 6.3(a) and Table 6.3(b) and were generally similar to those in recent years. Caesium-137 was positively detected at a low concentration in honey (as in recent years). Radionuclide concentrations were generally reported as less than values in 2023. Caesium-137 concentrations in sediment and soil are consistent with the distant effects of discharges from Sellafield, fallout from Chernobyl and nuclear weapons testing. Unlike in 2022, americium-241 concentrations were all reported as less than the detection limit in 2023.
Gamma dose rates measured in the surrounding area were difficult to distinguish from natural background (Table 6.3(b)). The tritium, gross alpha and gross beta concentrations were much lower than the investigation levels in the Water Supply (Water Quality) (Amendment) 2018 Regulations (retained from the European Directive 2013/51).
6.6 Holy Loch, Argyll and Bute
A small programme of monitoring at Holy Loch continued during 2023 to determine the effects of past discharges from the US submarine support facilities which closed in 1992. Radionuclide concentrations in the sediment sample collected in 2023 were low (Table 6.3(a)). Gamma dose rate measurements over intertidal areas (Table 6.3(b)) were higher than those values reported in recent years. The most recent habits survey to determine the consumption and occupancy rates by members of the public was undertaken in 1989 (Thurston & Gough 1992).
The external radiation dose to a person spending time on the loch shore was 0.011mSv in 2023, which was approximately 1% of the dose limit for members of the public of 1mSv and up from 0.006mSv in 2022 (Table 6.1). The increase in dose was mostly due to higher gamma dose rates measured over sediment at all 3 locations (Kilmun Pier, Mid-Loch and Robertsons Yard) in 2023.
In subsequent RIFE reports, dose assessments for the Holy Loch will no longer be undertaken. However, SEPA will continue to monitor the area under its Environmental Monitoring Programme, the results of which will be available. This decision has been taken on the basis that there are no ongoing discharges at the Holy Loch site and years of monitoring gives comfort that there is no realistic risk to the public using the area. Should results indicate that a dose assessment is needed, this will be undertaken.
6.7 Rosyth, Fife
The Rosyth naval dockyard is located on the north bank of the River Forth in Fife, 3km west of the Forth Road Bridge and some 50km from the mouth of the Firth of Forth. It is sited on reclaimed land, with reclamation completed in 1916. From 1916, the site was known as HM Dockyard Rosyth and activities conducted there included refitting and maintaining warships.
In 1997, Rosyth Royal Dockyard Limited (RRDL), a wholly owned subsidiary of Babcock International Group Marine Division, was set up to be responsible for the decommissioning of the dockyard site and the management of radioactive waste that had arisen from the re-fitting of nuclear submarines which ended in 2003. Site decommissioning started in 2006 and has mainly been completed, except for some small areas of the site where facilities are required to continue managing radioactive wastes.
The MOD sold the site to Babcock International Group Marine Division who now manage and operate the site. However, radioactive waste that was generated by the site, to support the nuclear submarine fleet, is owned by the MOD. Therefore, the MOD has entered into a contract with RRDL to manage all radioactive waste on the dockyard site. As the radioactive waste owner, the MOD maintains an overview of procedures to ensure RRDL fully complies with the terms and conditions of its contract.
In 2016, SEPA granted RRDL an authorisation (under RSA 93) to dispose of radioactive waste arising on the Rosyth dockyard site. This allows RRDL to dispose of LLW that arises from the decommissioning of the Rosyth premises, from former submarine re-fitting operations and from waste transferred from the MOD from the dismantling of the 7 redundant nuclear submarines currently stored afloat at the dockyard site. The authorisation was transitioned to a permit under the Environmental Authorisations (Scotland) Regulations 2018 (EASR18) with a new permit being issued in March 2019. In 2023, RRDL applied for a permit variation to allow the management of ILW from the dismantling of the 7 submarines stored at the dockyard. A Letter of Approval (LoA) (effective from 2016) to the MOD allows the transfer of LLW from the 7 nuclear submarines berthed at the Rosyth dockyard site to RRDL. Granting of the LoA and new authorisation to RRDL has permitted the start of the MOD submarine dismantling programme at Rosyth. Work to dismantle and remove radioactive and conventional wastes from each submarine and subsequently clean up the Rosyth site is expected to take up to 15 years to complete. The LoA is being transitioned to replicate an EASR18 permit in line with the recently revised Memorandum of Understanding between SEPA and the MOD.
The most recent habits survey was undertaken in 2022 (Scottish Environment Protection Agency no date). Copies of the most recent habits survey are available from SEPA by emailing [email protected].
The ‘total dose’ from all pathways and sources of radiation was 0.008mSv in 2023 (Table 6.1), which is less than 1% of the dose limit to members of the public. In 2023, the representative person was an adult consuming crustacean shellfish at high rates and unchanged from 2022. The increase in ‘total dose’ was mostly attributed to a higher limit of detection associated with the measurement of americium-241 in the winkle sample used in the assessment in 2023. The source specific assessment for marine pathways (fishermen and beach users) was estimated to be 0.006mSv in 2023 (an increase from less than 0.005mSv in 2022). The reason for the increase in dose was the same as that contributing to the maximum ‘total dose’.
The gaseous and liquid discharges from Rosyth in 2023 are given in Appendix 1 (Table A1.1 and Table A1.2, respectively), and solid waste transfers in Table A1.4. Liquid wastes are discharged via a dedicated pipeline to the Firth of Forth. Liquid discharges of tritium decreased in 2023 in comparison to that in 2022. During the reporting year a smaller percentage of the effluent discharged arose from the drain down of the submarine undergoing decommissioning. This accounted for the decrease in tritium discharged.
The direct radiation from the site was less than 0.001mSv in 2023 (Table 1.1). This was unchanged from 2022. This value of direct radiation is based upon actual measurements and a more representative background radiation measurement location.
SEPA’s routine monitoring programme included analysis of shellfish, environmental indicator materials and measurements of gamma dose rates in intertidal areas. Results are shown in Table 6.3(a). The radioactivity concentrations in freshwater measured were low in 2023, and similar to those observed in recent years, and in most part due to the combined effects of Sellafield, weapon testing and Chernobyl. Gamma dose rates were generally similar (in comparison to those observed in recent years) and difficult to distinguish from natural background.
6.8 Vulcan NRTE, Highland
The Vulcan Naval Reactor Test Establishment (NRTE) is operated by the Submarine Delivery Agency, part of the MOD, and its purpose was to prototype submarine nuclear reactors. It is located adjacent to the Dounreay site, and the impact of its discharges is considered along with those from Dounreay (in Section 5). The transfer of solid and aqueous waste to DSRL and the disposal of gaseous waste are made under a Letter of Approval (LoA) between SEPA and the MOD. Magnox Limited issued a statement regarding future decommissioning and stated: “Magnox, together with Dounreay, will be part of a joint working team exploring the option of transferring the future decommissioning of the MOD’s Vulcan site to the NDA Group. Any transfer would be subject to regulatory approval and sign-off by Government and is unlikely to take place before 2026”.
Gaseous discharges, and solid waste transfers, from Vulcan NRTE in 2023 are given in Appendix 1 (Table A1.1 and Table A1.4, respectively).
7 Industrial, landfill, legacy and other non-nuclear sites
Highlights
- doses (dominated by the effects of legacy discharges from other sources) increased at the LLWR in 2023
- doses at landfill sites were less than 0.5% of the dose limit in 2023
- doses (dominated by the effects of naturally occurring radionuclides from legacy discharges) increased at Whitehaven in 2023
This section considers the results of monitoring by the Environment Agency, FSA and SEPA for industrial, landfill, legacy and other non-nuclear sites that may have introduced radioactivity into the environment:
- the main disposal site for solid radioactive wastes in the UK, at the LLWR near Drigg in Cumbria, as well as a recycling facility and other landfill sites that received small quantities of solid wastes
- one legacy site in England, near Whitehaven, Cumbria, which was used to manufacture phosphoric acid from imported phosphate ore
- two legacy sites in Scotland, at Dalgety Bay, Fife, and Kinloss, Moray
- other non-nuclear sites
Data tables and where applicable, results of radiological dose assessments for these sites, in Open Document Spreadsheet (ODS) format are downloadable from the main RIFE page.
7.1 Low Level Waste Repository near Drigg, Cumbria
The LLWR is the UK’s national facility for the disposal of lower activity waste and is located on the west Cumbrian coast, southeast of Sellafield. The main function of the LLWR is to receive low activity solid radioactive wastes from all UK nuclear licensed sites (except Dounreay, where the adjacent disposal facility began accepting waste in 2015) and many non-nuclear sites. Where possible the waste is compacted, and then most waste is grouted within containers before disposal. Wastes are currently disposed of in engineered concrete vaults on land, whereas prior to the early 1990s waste was disposed of in open clay lined trenches. Work, expected to last 4 years, has begun on the final capping and vaults, which are ready for permanent closure.
The site is owned by the NDA and operated on their behalf by LLWR Limited. In 2018, the NDA awarded the incumbent PBO, UK Nuclear Waste Management Limited (UKNWM), a third (and final) contract for the management of LLWR Limited. In January 2022, NWS was launched. This brought together the operator of the LLWR, GDF developer Radioactive Waste Management Limited and the NDA group’s integrated waste management programmes into a single organisation. A five-year plan has been published setting out the long-term future of the site through to final closure, expected in 2129 (Low Level Waste Repository Limited 2018). LLWR’s Plutonium Contaminated Materials (PCM) Decommissioning Programme was completed in 2019, almost 4 years ahead of schedule. Five decommissioned concrete bunkers which housed legacy PCM, will be demolished and material re-used as in-fill for the final engineered cap over vaults and trenches.
The disposal permit allows for the discharge of leachate from the site through a marine pipeline. These discharges are small compared with those discharged from the nearby Sellafield site (Appendix 1). Marine monitoring of the LLWR is therefore subsumed within the Sellafield programme, described in Section 3.3. The contribution to exposures due to LLWR discharges is negligible compared with that attributable to Sellafield and any effects of LLWR discharges in the marine environment could not, in 2023 be distinguished from those due to Sellafield.
The current permit allows for continued solid radioactive waste disposal at the site, including permission to dispose of further radioactive waste beyond Vault 8, and limits disposals against a lifetime capacity for the site. In financial year 2017/18, the site commenced its long-term Repository Development Programme (RDP) (Low Level Waste Repository Limited 2018). In 2019, Revised Joint Waste Management Plans (JWMP) were published (in conjunction with LLW Repository Limited) for 3 radioactive waste-producing site licence companies (LLWR Limited, Magnox Limited and Sellafield Limited), covering the financial years, 2019/20 to 2023/24. More information can be found at the UK government’s website: joint waste management plans.
Waste received at the site will have a final disposal location allocated to it at the appropriate time, consequently, in the future, once the closure of Vault 8 has commenced as part of the RDP works, it is intended to report the quantity of solid radioactive waste finally disposed at the site. In the meantime, while development work progresses on the final waste disposal location and capping arrangements, Table A1.3 records, for the financial year 2023/24, both solid radioactive wastes already disposed in Vault 8 and the solid radioactive wastes accepted by the site (with the intention to dispose and currently stored within Vaults 8 and 9, pending disposal). A total of 624m3 of waste was received by the site with the intention of disposal in financial year 2023/24, bringing the cumulative total to 254,000m3. As started in 2016, the radiological data, given in Table A1.3, are recorded by financial year, instead of calendar year. All activities in terms of either disposal or receipt of solid radioactive waste with the intention of disposal have been within the lifetime capacity for the site.
Although the permit for routine disposal to the Drigg Stream has been revoked, reassurance monitoring has continued for samples of water and sediment. The results are given in Table 7.2. The tritium, gross alpha and gross beta concentrations in the stream were below the investigation levels for drinking water in the Water Supply (Water Quality) (Amendment) 2018 Regulations (retained from European Directive 2013/51). Although the stream is not known to be used as a source of drinking water, it is possible that occasional use could occur, for example by campers. If the stream was used as a drinking water supply for 3 weeks, the annual dose would be less than 0.005mSv. Concentrations of some radionuclides (plutonium-238 and plutonium-239+240) in sediment from the Drigg stream were similar to those in previous years. They reflect the legacy of direct discharges of leachate from the disposal site into the stream (Limited 2002). This practice stopped in 1991.
In the past, groundwater from some of the trenches on the LLWR site migrated eastwards towards a railway drain that runs along the perimeter of the site. Radioactivity from the LLWR was detected in the drain water. The previous operators of the site, British Nuclear Fuels plc (BNFL) took steps in the early 1990s to reduce migration of water from the trenches by building a ‘cut-off wall’ to reduce lateral migration of leachate. The results of monitoring in 2023 show that the activity concentrations have continued to be very low in the railway drain and have reduced significantly since the construction of the cut-off wall. Tritium, gross alpha and gross beta concentrations in the drain were also below the investigation levels for drinking water in the Water Supply (Water Quality) (Amendment) 2018 Regulations (retained from European Directive 2013/51).
The monitoring programme of terrestrial foodstuffs at the site was primarily directed at the potential migration of radionuclides from the waste burial site via groundwater, since the disposals of gaseous wastes are very small. Results for 2023 are given in Table 7.2 and these provide very limited evidence in support of the proposition that radioactivity in leachate from the LLWR might be transferring to foods. Concentrations of radionuclides were generally similar to (or lower than) those measured near Sellafield (Section 3.3). A new habits survey for LLWR near Drigg was undertaken in 2023 and the results have been included in the dose assessments for the site (Moore and others, 2024b).
The ‘total dose’ from all pathways and sources of radiation was 0.23mSv in 2023, or 23% of the dose limit for members of the public of 1mSv (Table 1.2 and Table 7.1) and includes a component due to the fallout from Chernobyl and nuclear weapons testing. This dose was dominated by the effects of naturally occurring radionuclides and the legacy of discharges into the sea at Sellafield, which are near to the LLWR site (see Section 3.3.1) and a smaller contribution from sources of direct radiation on site. If these effects were to be excluded, and the sources of exposure from the LLWR are considered, the ‘total dose’ from gaseous releases and direct radiation was 0.038mSv in 2023 (Table 1.2). The representative person was an adult living near the site, a change from 2022 (an infant living near the site). The increase in ‘total dose’ (from 0.030mSv in 2022, as amended in the RIFE 28 Errata) was mostly due to the revision of habits information. A source specific assessment of exposure for consumers of locally grown terrestrial food (animals fed on oats), using 2023 modelled activity concentrations in animal products, gives an exposure that was 0.007mSv in 2023, and similar to that in recent years.
7.2 Metals Recycling Facility, Lillyhall, Cumbria
The Metals Recycling Facility (MRF), operated by Cyclife UK Limited, is a small low hazard facility located at the Lillyhall Industrial Estate near Workington in Cumbria. The MRF receives metallic waste items contaminated with low quantities of radiological contamination from clients within the UK nuclear industry. These items are processed on a batch basis. Techniques used include size reduction (if required) using conventional hot and cold cutting methods, with subsequent decontamination using industrial grit blasting equipment.
The permit for the MRF site allows discharges of gaseous waste to the environment via a main stack and of aqueous waste to the sewer. Low discharge limits are set for both aqueous and gaseous discharges. Very small discharges were released during 2023 (Appendix 1, Table A1.1 and Table A1.2). The permit includes conditions requiring Cyclife UK Limited to monitor discharges and undertake environmental monitoring. As in recent years, direct radiation from the site was less than 0.001mSv in 2023 (Table 1.1) and the radiological impact was very low.
A direct radiation observation survey was undertaken in 2018 (Clyne 2021). This was the first habits survey to be carried out at the MRF and it was undertaken to ensure consistency with other nuclear licensed sites in the UK. The qualitative survey focussed on the area adjacent to the waste container park that had resulted in the elevated dose rates in 2016. Quantitative habits data were not obtained as the time spent by members of the public undertaking activities in the area was minimal.
7.3 Tradebe-Inutec, Winfrith, Dorset
The Tradebe-Inutec site is a radiological waste processing facility, for the wider nuclear industry, located adjacent to the Magnox Winfrith site. In early 2019, Tradebe-Inutec acquired buildings and land at Winfrith from the NDA and the ONR and Environment Agency granted a new nuclear site licence and environmental permit transfer (respectively) to Inutec Limited, who trade as Tradebe-Inutec. Prior to this, Tradebe-Inutec had been operating as a tenant of Magnox Limited, now Nuclear Restoration Services. The impact of its site operations and gaseous discharges is considered along with those from the Magnox Winfrith site (in Section 5.4).
Gaseous discharges from Tradebe-Inutec are also made via stacks to the local environment and are given in Appendix 1 (Table A1.1). As in 2022, discharges of alpha, carbon-14 and other radioelements were less than 1% of the discharge limits. The dose from direct radiation from the Tradebe-Inutec site is lower (0.001mSv) than the Magnox Winfrith site (Table 1.1).
Liquid waste from Tradebe-Inutec is transferred off site for disposal into Southampton Water under a non-nuclear permit (included in Table 7.10) and therefore, these impacts are not considered in the RIFE report.
7.4 Other landfill sites
Some organisations are granted permits by SEPA (in Scotland), the Environment Agency (in England) and NRW (in Wales) to dispose of solid wastes containing low quantities of radioactivity to approved landfill sites. In Northern Ireland, this type of waste is transferred to Great Britain for incineration. Waste with very low quantities of radioactivity can also be disposed of in general refuse. Radioactivity in wastes can migrate into leachate and in some cases can enter the groundwater. SEPA and the Environment Agency carry out monitoring of leachates. The locations of landfill sites considered in 2023 are shown in Figure 7.1 and the results are presented in Table 7.3 and Table 7.4, alongside the respective regions and locations.
Figure 7.1. Landfill sites monitored in 2023.
The results, in common with previous years, showed evidence for migration of tritium from some of the disposal sites. The reported tritium concentrations vary from year to year. The variation is thought to be related to changes in rainfall quantity and resulting leachate production and the use of different boreholes for sampling. A possible source of the tritium is thought to be due to disposal of Gaseous Tritium Light Devices (Mobbs, Barraclough & Napier 1998). As in recent years, inadvertent ingestion of leachate (2.5l per year) from the Summerston landfill (City of Glasgow) site (with the highest observed concentration of tritium) would result in a dose of less than 0.005mSv in 2023 (Table 7.1), or less than 0.5% of the dose limit for members of the public of 1mSv. Similarly, the annual dose from ingestion of uranium isotopes in leachate from Clifton Marsh was also less than 0.005mSv in 2023.
In 2007, the UK government introduced a more flexible framework for the disposal of certain categories of LLW to landfill. Further details and information are provided on the website: managing the use and disposal of radioactive and nuclear substances and waste.
In England and Wales, disposal of LLW at landfill sites requires both landfill companies and nuclear operators to hold permits to dispose of LLW and very low-level waste (VLLW). The 2007 government policy led to applications from landfill operators for permits to dispose of LLW at their sites. The landfill sites were:
- Waste Recycling Group Limited (part of FCC Environmental) at the Lillyhall Landfill Site in Cumbria. Their permit, issued in 2011, allows disposal of VLLW.
- Augean at the East Northants Resource Management Facility (ENRMF), near Kings Cliffe, Northamptonshire. Their permit, issued in 2016, allows the disposal of low activity LLW and VLLW. This permit also requires the operator to carry out periodic environmental monitoring. The results and techniques used are annually audited by the Environment Agency.
- Suez Recycling and Recovery UK Limited (formerly SITA UK) at Clifton Marsh in Lancashire. A permit to dispose of LLW was issued by the Environment Agency in 2012.
Disposals of LLW at Clifton Marsh have continued under the new permitting arrangements.
Disposals of LLW at the ENRMF landfill site, near Kings Cliffe, began in 2011 and were from non-nuclear site remediation works. The first consignment from a nuclear licensed site was in 2012. This comprised soil, concrete, rubble and clay pipes from the drains on the Harwell site. In parallel, the Environment Agency began a programme of monitoring within and around the ENRMF site to provide a baseline and allow detection of any future changes (for example (Environment Agency, Food Standards Agency, Natural Resources Wales and others, 2014)). In 2022, samples were taken, filtered and analysed for radiological composition from groundwater boreholes and off-site watercourses. Both the filtrate and the particulate were analysed for their radioactivity content, along with some bulk water samples. The results are given in Table 7.5. The results were generally reported as less than values. Naturally occurring radionuclides were present at values expected due to natural sources. Gross alpha and gross beta concentrations in off-site watercourses were below the investigation levels for drinking water in the Water Supply (Water Quality) (Amendment) 2018 Regulations (retained from the European Directive 2013/51) of 0.1 and 1.0Bq l-1, respectively. No use of water for drinking has been observed. Where sampling was repeated, the results were similar to those in previous years. Based on inadvertent ingestion of borehole or surface water at concentrations presented in Table 7.5, the dose in 2023 was estimated to be less than 0.005mSv, or less than 0.5% of the dose limit for members of the public of 1mSv (Table 7.1). The assessment excludes potassium-40 because its presence is homeostatically controlled in the body.
After receiving a permit variation application from FCC Recycling (UK) Limited and subsequent consultation with the local public, professional bodies and stakeholders in 2019, the EA issued a permit variation in July 2021. This allows the site to receive radioactive waste at up to a maximum average activity of 200Bq g-1 (previously, a maximum average activity of 4Bq g-1, or 40Bq g-1 for tritium). For further information, please visit the following website: (Environment Agency lillyhall landfill site rsa permit variation consultation).
SEPA’s monitoring programme at the Stoneyhill Landfill Site in Aberdeenshire, authorised to dispose of conditioned NORM waste, ceased in 2016. Results up to 2015 are included in earlier RIFE reports and show no significant radiological impact (for example (Environment Agency, Food Standards Agency and others, 2016)).
NORM is found within oil and gas reserves and is consequently extracted along with the oil and gas. The NORM can precipitate onto oil and gas industry equipment creating an insoluble scale (NORM scale). The presence of this scale reduces the efficiency of the equipment and must be removed. Suez Recycling and Recovery UK Limited, the operators of the Stoneyhill Landfill site, has constructed a descaling facility adjacent to the landfill in partnership with Nuvia Limited. This facility descales oil and gas industry equipment, such as pipes, using pressurised water. The solid scale removed from the equipment is then grouted into drums and can be consigned to Stoneyhill Landfill site in accordance with the authorisation granted in 2012.
7.5 Past phosphate processing, Whitehaven, Cumbria
An important historical man-made source of naturally occurring radionuclides in the marine environment was the chemical plant near Whitehaven in Cumbria, which used to manufacture phosphoric acid (for use in detergents) from imported phosphate ore (Rollo and others, 1992). Processing of ore resulted in a liquid waste slurry (phosphogypsum) containing most of the thorium, uranium and radioactive decay products (including polonium-210 and lead-210) originally present in the ore, and this was discharged by pipeline to Saltom Bay.
The slurry is regarded as TENORM, meaning that, elevated levels of NORM resulting from industrial activity. Historical discharges continue to have an impact (close to the former discharge point), through the production of the radioactive products. The impact is due to the decay of long-lived parent radionuclides previously discharged to sea. Both polonium-210 and lead-210 are important radionuclides in that small changes in activity concentrations above background significantly influence the dose contribution from these radionuclides. This is due to their relatively high dose coefficient used to convert intake of radioactivity into a radiation dose.
Processing of phosphoric acid at the plant ceased at the end of 2001. The plant was subsequently decommissioned and the authorisation to discharge radioactive wastes was revoked by the Environment Agency.
The results of routine monitoring for naturally occurring radioactivity near the site in 2023 are shown in Table 7.6. Routine analytical effort is focused on polonium-210 and lead-210, which concentrate in marine species and are the important radionuclides in terms of potential dose to the public. As in previous years, polonium-210 and other naturally occurring radionuclides were slightly enhanced near Whitehaven but quickly reduced to background values further away. Figure 7.2 to Figure 7.4 show how concentrations of polonium-210 in winkles, crabs and lobsters have generally decreased since 1998, with larger concentrations variations in lobsters since 2014. Concentrations in the early 1990s were in excess of 100Bq kg-1 (fresh weight), when discharges were higher, prior to a change in site operations (in 1992) and later plant closure in 1997. There were some small variations in concentrations of polonium-210 in local samples in 2023 (where comparisons can be made), in comparison with those in 2022. Polonium-210 concentrations were generally higher in both crab and lobster samples in 2023, and as in recent years, these concentrations continued to be within or close to the expected range due to natural sources. For crustacean and other seafood samples, it is now difficult to distinguish between the measured radionuclide concentrations and the range of concentrations normally expected from naturally sourced radioactivity. The latter are shown in Figure 7.2 to Figure 7.4 and in Appendix 6 (minimum, median and maximum). There were small enhancements for some samples at other locations above the expected natural background median values for marine species, however, the majority were within the ranges observed in the undisturbed marine environment. It is considered prudent to continue to estimate doses at Whitehaven whilst there remains an indication that concentrations are higher than natural background. Further analysis has confirmed that this approach is unlikely to underestimate doses (Dewar and others, 2014).
Figure 7.2. Polonium-210 discharge from Whitehaven and concentration in winkles at Parton, 1990 to 2023.
Figure 7.3. Polonium-210 discharge from Whitehaven and concentration in crabs at Parton, 1990 to 2023.
Figure 7.4. Polonium-210 discharge from Whitehaven and concentration in lobsters at Parton, 1990 to 2023.
In 2018, the Environment Agency, with the support of the FSA, NIEA and SEPA, performed additional polonium-210 analyses in shellfish samples to obtain baseline data, providing naturally sourced polonium-210 concentrations that are unlikely to be influenced by TENORM in the Irish Sea. Further details are presented in RIFE 24 (Environment Agency, Food Standards Agency, Food Standards Scotland, Natural Resources Wales and others,. 2019).
The exposure pathway considered for the assessment at Whitehaven was internal irradiation, due to the ingestion of naturally occurring radioactivity in local fish and shellfish. The representative person was a Cumbrian coastal community consumer who, centred on the Sellafield site to the south of Whitehaven, obtained their sources of seafood from locations such as Whitehaven, Nethertown and Parton. This consumer is also considered in the assessment of the marine impacts of the Sellafield and LLWR (near Drigg) sites (Sections 3.3 and 7.1). The estimated contribution due to background median concentrations of naturally occurring radionuclides is subtracted from the measured activity concentration. Consumption rates for people who eat seafood at high rates were reviewed and revised in 2023 (Moore and others, 2024a). Revised figures for consumption rates, together with occupancy rates, are provided in Appendix 4 (Table A4.2). The dose coefficient for polonium-210 is based on a value of the gut transfer factor of 0.5 for all foods.
The ‘total dose’ to a local high-rate consumer of seafood was 0.23mSv in 2023 (Table 7.1), or 23% of the dose limit to members of the public. This is a decrease from 0.24mSv in 2022. The dose includes the effects of all sources near the site: technically enhanced naturally occurring radionuclides from the non-nuclear industrial activity (in other words, TENORM) and Sellafield operations. The contribution to the ‘total dose’ from enhanced natural radionuclides was 0.21mSv and was lower in 2023, in comparison to that in 2022 (0.22mSv). The decrease in ‘total dose’ in 2023 was mostly attributed to revision of habits information particularly the reduced consumption rate of both fish and crustacean species. The largest contribution to dose to a Cumbrian coastal community seafood consumer near Whitehaven and Sellafield continues to be from the legacy of historical discharges near Whitehaven. A source specific dose assessment targeted directly at local consumers of seafood (at high rates), gives an exposure of 0.36mSv in 2023 (Table 7.1).
The longer-term trend in annual ‘total dose’ over the period 2012 to 2023 is shown in Figure 7.5. The variations in ‘total dose’ over the period 2012 to 2023 reflect changes in polonium-210 concentrations, consumption rates and the range of seafood species consumed by individuals at high-rates, including that of crustaceans. Over a longer period, the trend is of generally declining dose (Figure 7.4, (Environment Agency, Food Standards Agency, Food Standards Scotland, Natural Resources Wales and others, 2018)).
Figure 7.5. Trend in ‘total dose’ to seafood consumers from naturally- occurring radionuclides near Whitehaven, 2012 to 2023. Data are presented to 2 significant figures.
Year | Dose, mSv y-1 |
---|---|
2012 | 0.22 |
2013 | 0.021 |
2014 | 0.15 |
2015 | 0.35 |
2016 | 0.34 |
2017 | 0.18 |
2018 | 0.33 |
2019 | 0.20 |
2020 | 0.25 |
2021 | 0.19 |
2022 | 0.22 |
2023 | 0.21 |
7.6 Former military airbase, Dalgety Bay, Fife
Radioactive items containing radium-226 and associated decay products have been detected at Dalgety Bay in Fife since at least 1990. The contamination is associated with historical disposals of waste from past military operations at the Royal Naval Air Station (RNAS) Donibristle, which closed in 1959 and upon which large areas of the town of Dalgety Bay have been built. The air station played a role as an aircraft repair, refitting and salvage yard. It is believed that waste was incinerated, and the resultant ash and clinker was disposed of by reclaiming land from the sea. Following years of erosion at the site the contamination is being exposed on and adjacent to the foreshore. Some of the incinerated material contained items such as dials and levers which had been painted with luminous paint containing radium-226.
In 1990, environmental monitoring showed elevated activity concentrations in the Dalgety Bay area. The monitoring was undertaken as part of the routine environmental monitoring programme for Rosyth Royal Dockyard Limited conducted in accordance with the dockyard’s authorisation to dispose of liquid radioactive effluent to the Firth of Forth. Some material was removed for analysis, which indicated the presence of radium-226. Further investigation confirmed that the contamination could not have originated from the dockyard and was most likely to be associated with past practices related to the nearby former RNAS Donibristle/HMS Merlin military airfield. Since this initial discovery, there have been several monitoring exercises to determine the extent of this contamination. In 2017, SEPA issued guidance on monitoring for heterogeneous radium-226 sources resulting from historic luminising activities or waste disposal sites (Scottish Environment Protection Agency 2017).
Additional public protection measures were established following the increased number of particles and the discovery of some high activity particles in 2011. These were maintained between 2020 and 2024. A monthly beach monitoring and particle recovery programme was adopted in 2012 by a contractor working on behalf of the MOD and this remains in place. The information signs advising the public of the contamination and precautions to be taken remain in place. In addition, the FEPA Order issued by FSS (then FSA in Scotland) prohibiting the collection of seafood from the Dalgety Bay area remains in force. SEPA undertook a one-year programme of shellfish monitoring from February 2012 during which no particles were detected in the shellfish. All shellfish samples collected were analysed for the presence of radium-226 and all were reported as less than values. During routine monitoring of mussel beds in 2015 a particle was detected in this area (for the first time since 2011) and retrieved, indicating that the continuation of these protection measures is reducing the risks to members of the public whilst further work continues to address the contamination.
The Committee on Medical Aspects of Radiation in the Environment (COMARE) recommended that effective remediation of the affected area be undertaken as soon as is possible. This recommendation followed the publication of the risk assessment in 2013, which was considered alongside the Appropriate Person Report. This Appropriate Person Report included a comprehensive study of the land ownership and history at Dalgety Bay. The COMARE recommendation, amongst others, was subsequently published in 2014 in COMARE’s 15th report. The MOD has progressed with addressing the contamination by initially publishing its Outline Management Options Appraisal Report in 2014, followed by a further publication in 2014 of its broad management strategy and timescale for implementation of its preferred management option. Copies of these reports are available on the UK government website: committee on medical aspects of radiation in the environment.
The environmental impact assessment (EIA) in support of the planning application for the remediation works was submitted to Fife Council for consideration. In 2017, the planning application for the remediation works was submitted to Fife Council and subsequently approved.
The remediation contract was awarded by MOD in February 2020 and an EASR18 permit to undertake the required work was granted by SEPA in May 2021. Remediation work took place between 2021 and 2023. The works involved extraction of beach material which was then transferred to a compound where the material was screened for asbestos and then radioactive contamination on a conveyor system.
Asbestos material and oversized material were monitored for radioactive contamination separately by hand.
Screened material was then replaced onto the beach. This was covered with a geotextile membrane on top of which graded rock armour was fixed in place.
It is estimated that a total of over 13,000 items have been removed from the beach as a result of both the remediation works and previous monitoring undertaken since 1990.
Verification monitoring will be undertaken following completion of the works for at least 2 years. By analysing the activity range and depth of particles recovered from the area, SEPA will determine if the remediation objective has been met. Following this the site will then be regularly monitored by SEPA (radiological monitoring) and Fife Council (rock armour assessment).
Further details on the work at Dalgety Bay can be found on the Radioactive Substances pages on SEPA’s website: SEPA Dalgety Bay.
7.7 Former military airbase, Kinloss Barracks, Moray
Radioactive items containing radium-226 and associated decay products have been detected on an area of land which used to form part of the former RAF Kinloss, now Kinloss Barracks. The contamination is associated with historical disposals of waste from past military operations at the site resulting from the dismantling of aircraft no longer required by the RAF following World War II. During the late 1940s, the aircraft were stripped for their scrap metal, with the remains being burnt and/or buried at the site. The source of the radium-226 and associated decay products are the various pieces of aircraft instrumentation which were luminised with radium paint.
SEPA has undertaken monitoring surveys at the site which positively identified the presence of radium-226 and has published an assessment of the risks posed to the public (Natural Scotland & Scottish Environment Protection Agency 2016). Currently, the site is largely undeveloped open land covered in gorse, with several wind turbines and access tracks. The area has a few informal paths crossing the land that is used by visitors and dog walkers. The contamination detected at the site is all currently buried at depth. Current uses of the site do not involve intrusion into the ground to any significant depth; thus, there is no current pathway for exposure via skin contact, ingestion or inhalation. Exposure via external gamma irradiation is possible but is significantly below the relevant dose criteria detailed in the Radioactive Contaminated Land Statutory Guidance (Scottish Executive 2006; Scottish Government 2009).
The risk assessment of the series of monitoring surveys concluded that, under its current use, there are no viable or credible exposure pathways for the public to be exposed to the contamination and that this site does not currently meet the definition of radioactive contaminated land (Natural Scotland & Scottish Environment Protection Agency 2016). However, SEPA will keep this site under review as a change in land use on the site may alter the potential exposure pathways. To access the full risk assessment report please visit the radioactive substances pages available on SEPA’s website: www.sepa.org.uk.
7.8 Other non-nuclear sites
Small quantities of gaseous and liquid radioactive wastes are routinely discharged from a wide range of other non-nuclear sites in the UK on land (including to the atmosphere from industrial stacks and incinerators), and from offshore oil and gas installations.
A summary of the most recent data for the quantities discharged under regulation for England and Northern Ireland in 2023 is given in Table 7.7 and Table 7.8. Data for Scotland are presented in Table 7.9 and Table 7.10 in terms of OSPAR regions (Region II represents the Greater North Sea and Region III the Celtic Sea). Data for Wales are presented in Table 7.11. This change in format allows easier trend analysis to be performed for OSPAR. The data are grouped according to the main industries giving rise to such wastes in the UK and exclude information for other industries considered in other sections of this report, principally the nuclear sector. The main industries are:
- oil and gas, both off and onshore
- education, including universities and colleges
- hospitals
- other, which includes research, manufacturing and public sector
Discharges may also occur without an authorisation or permit when the quantities are below the need for specific regulatory control. For example, discharges of natural radionuclides are made from coal-fired power stations because of the presence of trace quantities of uranium and thorium and their decay products in coal (Corbett 1983).
As indicated in Section 1, general monitoring of the British Isles as reported elsewhere in this report has not detected any gross effects from non-nuclear sources. Occasionally, routine programmes directed at nuclear licensed site operations detect the effects of discharges from the non-nuclear sector and, when this occurs, a comment is made in the relevant nuclear licensed site text. The radiological impact of the radioactivity from the non-nuclear sector detected inadvertently in this way is very low.
Monitoring of the effect of the non-nuclear sector is limited because of the relatively low impact of the discharges. However, programmes are carried out to confirm that impacts are low and, when these occur, they are described in this report.
The Pharmaron UK radiolabelling facility in Cardiff, discharges tritium and carbon-14 to the atmosphere and to the marine environment, via the Cardiff sewage treatment works outfall. This site began operation after Quotient Bioresearch (Radiochemicals) Ltd in 2010 (later becoming Pharmaron UK in 2017) acquired GE Healthcare’s custom radiolabelling division which previously operated at the Maynard Centre in Cardiff (previously reported in RIFE (Environment Agency, Food Standards Agency and others, 2012)). These operations were then moved to new premises in Cardiff, under a non-nuclear permit with lower discharge limits. Discharges from the Pharmaron UK facility are included in the discharges reported in Table 7.11. The radiological impacts of these discharges are low.
Following a notification of elevated environmental levels of carbon-14 at Cowpen Bewley, near Billingham, the Environment Agency have reviewed available evidence and concluded that the most likely source is past permitted discharges from BlyChem Ltd to Cowbridge Beck via Billingham Sewage Treatment Works (STW). The annual discharges of carbon-14, via this discharge route, have declined by a factor of 10 over the last 20 years and in 2008 the Billingham STW discharge point was diverted to discharge to the North Sea via a pipeline over 3km offshore from Seaton Carew. An infrequently used storm overflow route remains into Cowbridge Beck. Given the relatively long half-life of carbon-14, the Environment Agency expect a slow but gradual decline of carbon-14 levels in this area through environmental dilution and dispersion processes. The Environment Agency have introduced routine carbon-14 monitoring of sediment at one location in Greatham Creek to provide re-assurance that this understanding is correct. These additional concentration data are reported in Table 7.12.
In 2023, SEPA continued to undertake a small-scale survey (as part of the annual programme) of the effects of discharges from non-nuclear operators by analysing mussel samples and other materials from the River Clyde, the Firth of Forth and sludge pellets from a sewage treatment works (at Daldowie). The results are given in Table 7.13. The results in 2023 were generally similar to those in 2022. Activity concentrations were typical of the expected effects from Sellafield discharges at this distance and the presence of iodine-131 in sludge pellets (probably from a hospital source). An assessment was undertaken to determine the dose to the representative high-rate mollusc consumer. The dose was estimated to be less than 0.005mSv in 2023, or approximately 0.5% of the dose limit for members of the public, and unchanged from 2022.
Scotoil, in Aberdeen City, operates a cleaning facility for equipment from the oil and gas industry contaminated with enhanced concentrations of radionuclides of natural origin. The facility is authorised to discharge liquid effluent to the marine environment within the limitations and conditions of the authorisation, which includes limits for radium-226, radium-228, lead-210 and polonium-210 discharges. The authorisation includes conditions requiring Scotoil to undertake environmental monitoring. Prior to their operations, a fertiliser manufacturing process was operated on the site and made discharges to sea. Monitoring of seaweed (‘Fucus vesiculosus’) from Nigg Bay, near Aberdeen Harbour was carried out in 2023 and are reported in Table 3.11. In 2023, the dose rate on sediment was 0.068µGy h-1 and similar to background.
8 Regional monitoring
Highlights
- doses for the representative person were approximately 1% (or less) of the annual public dose limit in 2023
Regional monitoring in areas remote from nuclear licensed sites continued in 2023:
- to establish long distance transport of radioactivity from UK and other nuclear licensed sites
- to indicate general contamination of the food supply and the environment
- to provide data under UK obligations under the OSPAR Convention
The routine component parts of this programme are: sampling of seafood and environmental samples from the Channel Islands and Northern Ireland; monitoring UK ports of entry for foodstuffs from Japan and for other non-specific contamination; sampling of the UK food supply, air, rain, sediments, drinking water and seawater. Data tables and the detailed results of the dose assessments, in Open Document Spreadsheet (ODS) format, are downloadable from the main RIFE page.
8.1 Channel Islands
Samples of marine environmental materials were provided by the Channel Island States and measured for a range of radionuclides. The programme monitors the effects of radioactive discharges from the French reprocessing plant at La Hague and the power station at Flamanville. It also monitors any effects of historical disposals of radioactive waste in the Hurd Deep, a natural trough in the western English Channel. Fish and shellfish are monitored to determine exposure from the internal radiation pathway, and sediment is analysed for external exposures. Seawater and seaweeds are sampled as environmental indicator materials, and, in the latter case, because of their use as fertilisers. A review of marine radioactivity in the Channel Islands from 1990 to 2009 has been published (Hughes, Runacres & Leonard 2011).
The results of monitoring for 2023 are given in Table 8.1, as in 2022, no samples were collected or analysed from Alderney. There was evidence of routine releases from the nuclear industry in some food and environmental samples (for example, technetium-99). However, activity concentrations in fish and shellfish were low and similar to those in previous years. It is generally difficult to attribute the results to different sources, including fallout from nuclear weapons testing, due to the low values detected. No evidence for significant releases of activity from the Hurd Deep site was found.
In 2023, the dose to the representative person, consuming large amounts of fish and shellfish was estimated to be less than 0.005mSv, or less than 0.5% of the dose limit for members of the public. The assessment included a contribution from external exposure. The concentrations of artificial radionuclides in the marine environment of the Channel Islands and the effects of discharges from local sources, therefore, continued to be of negligible radiological significance.
The collection of milk and crop samples from the Channel Island States ceased in 2014. Results up to 2013 are included in earlier RIFE reports (for example (Environment Agency and others, 2013)) and the data indicated no significant effects from UK or other nuclear installations.
8.2 Isle of Man
The Environment Agency has carried out a review of its environmental monitoring on the Isle of Man. Following this review, the Environment Agency’s marine monitoring on the Isle of Man ceased in 2016. Results up to 2015 are included in earlier RIFE reports (for example (Environment Agency, Food Standards Agency, Scotland and others, 2016)). Previous results have demonstrated that there has been no significant impact on the Isle of Man from discharges to sea from mainland nuclear installations in recent years.
The Government of the Isle of Man undertakes their own independent radioactivity monitoring programme and provides an indication of the far-field effects of current and historical discharges from Sellafield and other UK nuclear sites. These are reported annually: Isle of Man environmental radioactivity
8.3 Northern Ireland
The NIEA monitors the far-field effects of liquid discharges from Sellafield into the Irish Sea. The programme involved sampling fish, shellfish, and indicator materials from a range of locations along the coastline (Figure 8.1). The sample species/type and locations are listed in the respective site tables. Gamma dose rates were measured over intertidal areas to assess the external exposure pathway. The results of monitoring are given in Table 8.2(a) and Table 8.2(b).
Figure 8.1. Monitoring locations in Northern Ireland, 2023.
In 2023, the main effect of discharges from Sellafield was observed in concentrations of technetium-99 in shellfish and seaweed samples. These were similar to values reported in recent years, reflecting the considerably decreased inputs to the Irish Sea (see also Section 3.3.3). Caesium-137 concentrations were low and generally similar to those in 2022 and over the period 2003 to 2023. As expected, low concentrations of transuranic radionuclides were also detected in 2023. Reported concentrations are less than those found nearer to Sellafield and continued to be low, as in recent years (Figure 8.2). Further information on the trends in radioactivity in the marine environment of Northern Ireland has been published (Ly and others, 2015). The gamma dose rates over intertidal areas were similar to those in previous years.
Figure 8.2. Concentrations of americium-241 and caesium-137 in coastal sediments in Northern Ireland, 2002 to 2023.
A survey of consumption and occupancy in coastal regions of Northern Ireland established the habits of people consuming large quantities of fish and shellfish (Smith and others, 2002). Based on the monitoring results from the marine environment in 2023, the annual dose from the consumption of seafood and exposure over intertidal areas was 0.007mSv (Table 3.15), or less than 1% of the dose limit for members of the public.
Monitoring results for the terrestrial environment of Northern Ireland are included in the following parts of Section 8.
8.4 General diet
As part of the UK government and devolved administrations’ general responsibility for food safety, concentrations of radioactivity are determined in regional diets. These data (and data on other dietary components in Sections 8.5 and 8.6) previously formed the basis of the UK submission to the EC under Article 36 of the Euratom Treaty. While these data are no longer supplied to the EC for England, Wales and Northern Ireland, they will continue to be published in the RIFE reports.
In 2023, the concentrations found in a survey of radioactivity in canteen meals collected across the UK, and mixed diets in Scotland, were very low or typical of natural sources (Table 8.3). Activity concentrations were generally similar to those in previous years.
8.5 Milk
The programme of milk sampling across dairies in the UK continued in 2023. The aim is to collect and analyse samples on a monthly basis, for their radionuclide content. This programme provides useful information with which to compare data from farms close to nuclear licensed sites and other establishments that may enhance values above background activity concentrations. Prior to the UK’s exit from the EU, concentrations of radioactivity in the general diet were reported to the EC by the FSA (for England, Northern Ireland and Wales), and by SEPA (for Scotland). While these data are no longer supplied to the EC for England, Wales, and Northern Ireland, they will continue to be published in the RIFE reports.
The results of milk monitoring for 2023 are summarised in Table 8.4. Most results were similar to those in previous years (where comparisons can be made). The mean carbon-14 concentrations in England, Northern Ireland, Wales, and Scotland were all close to the expected background concentration in milk (see Appendix 6). The maximum concentrations of carbon-14 in milk for England (Devon), Northern Ireland (Co. Antrim), Wales (Gwynedd), and Scotland (Midlothian) were 26, 21, 12 and less than 16Bq l-1, respectively. As in previous years, tritium concentrations were reported as less than values at all remote sites. In 2023, strontium-90 concentrations were reported as less than values (or just above the less than value), and the mean concentration over the UK was less than 0.033Bq l-1 in 2023 (0.033Bq l-1 in 2022). In the past, the highest concentrations of radiocaesium in milk were from those regions that received the greatest amounts of fallout from Chernobyl. However, the concentrations are now very low, and it is not possible to distinguish this trend.
The radiation dose from consuming milk at average rates was assessed for various age groups. In 2023, the most exposed age group was infants (1-year-old). For the range of radionuclides analysed, the annual dose was less than 0.005mSv or less than 0.5% of the dose limit. Previous surveys (for example, (Food Standards Agency & Scottish Environment Protection Agency 2002)) have shown that if a full range of nuclides were to be analysed and assessed, the dose would be dominated by naturally occurring lead-210 and polonium-210, and artificial radionuclides would contribute less than 10% of the dose.
8.6 Crops
The programme of monitoring naturally occurring and artificial radionuclides in crops (in England, Wales and the Channel Islands) as a check on general food contamination (remote from nuclear sites) ceased in 2014. Further information on previously reported monitoring is available in earlier RIFE reports (for example (Environment Agency, Food Standards Agency, Natural Resources Wales and others, 2014)).
8.7 Airborne particulate, rain, freshwater and groundwater
Radioactivity in rainwater and air was monitored at several UK locations as part of the programme of background sampling managed by the Environment Agency and SEPA. These data are collected on behalf of the DESNZ, NIEA and, the Scottish and Welsh Governments. The results of monitoring are given in Table 8.5. The routine programme is comprised of two components:
- regular sampling and analysis on a quarterly basis
- supplementary analysis on an ‘ad hoc’ basis
Tritium and caesium-137 concentrations in air and rainwater are reported as less than values in 2023. Caesium-137 concentrations in air, as in recent years, remain less than 0.01% of those observed in 1986, the year of the Chernobyl reactor accident.
Concentrations of beryllium-7, a naturally occurring radionuclide formed by cosmic ray reactions in the upper atmosphere, were positively detected at similar values at all sampling locations. Peak air concentrations of this radionuclide tend to occur during spring and early summer, as a result of seasonal variations in the mixing of stratospheric and tropospheric air (Environment Agency 2002). Activity concentrations of the radionuclides reported in air and rainwater were very low and do not currently merit radiological assessment.
Sampling and analysis of freshwater from drinking water sources throughout the UK continued in 2023 (Figure 8.3). The sample locations and regions are listed in the respective site tables. These water data are collected by the Environment Agency (for England and Wales), NIEA (for Northern Ireland) and SEPA (for Scotland). Sampling was designed to represent the main drinking water sources, namely reservoirs, rivers and groundwater boreholes. Most of the water samples were representative of natural waters before treatment and supply to the public water system.
Figure 8.3. Drinking water sampling locations, 2023.
The results are given in Table 8.6 to Table 8.8 (inclusive). Tritium concentrations were all substantially below the investigation level for drinking water of 100Bq l-1 in the Water Supply (Water Quality) (Amendment) 2018 Regulations (retained from European Directive 2013/51) (where applicable) and all are reported as less than values (except for 2 results). At Gullielands Burn (Table 8.6), which is near to the Chapelcross nuclear licensed site, the tritium concentration was 6.9Bq l-1 in 2023 (similar to that in recent years).
The mean annual dose from consuming drinking water in the UK was 0.012mSv in 2023 (Table 8.9), and lower than the mean annual dose in 2022 (0.027mSv). The highest annual dose was estimated to be 0.015mSv for drinking water from Matlock, Derbyshire. The estimated doses were dominated by naturally occurring radionuclides and are generally similar to those in recent years. The annual dose from artificial radionuclides in drinking water was less than 0.001mSv.
Collection and analysis of groundwater samples from across Scotland was not performed in 2023. Results up to 2019 are included in earlier RIFE reports (for example, (Environment Agency and others,2020)).
8.8 Overseas incidents
Two overseas accidents have had direct implications for the UK: Chernobyl (1986) and Fukushima Dai-ichi (2011). Earlier RIFE reports have provided detailed results of monitoring by the environment agencies and the FSA (Environment Agency, Food Standards Agency and others, 2012).
For Chernobyl, the main sustained impact on the UK environment was in upland areas, where heavy rain fell in the days following the accident, but activity concentrations have now reduced substantially. The results of monitoring and estimated doses to consumers are available in earlier RIFE reports.
The accident at the Fukushima Dai-ichi nuclear power station in Japan in March 2011 resulted in significant quantities of radioactivity being released into the air and sea. Controls on imported food and animal feed products from Japan were implemented in 2011 (revised in 2016 and 2019). These controls were revoked in June 2022 for Great Britain (England, Wales and Scotland).
In Northern Ireland, European Regulations continue to apply under the terms of the UK’s withdrawal agreement from the EU. In September 2021, the EU published Commission Implementing Regulation (EU) 2021/1533 (European Commission 2022) which replaced Regulation 2016/6 in the EU. The EU retained enhanced controls on any food where there is a single instance of exceeding the maximum level of 100Bq kg-1, or similar. As a result, some controls will remain in place for food imported into the EU and Northern Ireland. The list of foods covered by the enhanced controls in the EU regulations is now very limited and includes wild mushrooms, foraged foods, and some species of fish.
A full description of the legislation, requirements and procedures involved for imports are provided in earlier RIFE reports (Environment Agency, Food Standards Agency and others, 2021).
Screening instruments are used at importation points of entry to the UK as a general check on possible contamination from unknown sources. In 2023, these instruments were not triggered by a food consignment at any point of entry into the UK.
8.9 Seawater surveys
The UK government and devolved administrations are committed to preventing pollution of the marine environment from ionising radiation, with the main aim of reducing concentrations in the environment to near background values for naturally occurring radioactive substances, and close to zero for artificial radioactive substances (Department for Business Energy and Industrial Strategy 2018). Therefore, a programme of surveillance into the distribution of important radionuclides is maintained using research vessels and other means of sampling.
The seawater surveys reported here also support international studies concerned with the quality status of coastal seas. The programme of radiological surveillance work provided the source data and, therefore, the means to monitor and assess progress in line with the UK’s commitments towards OSPAR’s 1998 Strategy for Radioactive Substances target for 2020 (part of the North-East Atlantic Environment Strategy adopted by OSPAR for the period 2010 to 2020), see Section 1.3.2 of this report for more details. The surveys also provide information that can be used to distinguish different sources of artificial radioactivity (for example, (Kershaw & Baxter 1995)) and to derive dispersion factors for nuclear licensed sites (for example, (Baxter & Camplin 1994)). In addition, the distribution of radioactivity in seawater around the British Isles is a significant factor in determining the variation in individual exposures at coastal sites, as seafood is a major contribution to food chain doses.
The research vessel programme on radionuclide distribution currently comprises annual surveys of the Bristol Channel/western English Channel and biennial surveys of the Irish Sea and the North Sea. The results obtained in 2023 are given in Figure 8.4 to Figure 8.8 and are discussed in the text below.
Figure 8.4. Concentrations (Bq l-1) of caesium-137 in surface water from the Irish Sea, September 2023
Figure 8.5. Concentrations (Bq l-1) of caesium-137 in surface water from the English Channel, March – April 2023.
Figure 8.6. Concentrations (Bq l-1) of tritium in surface water from the Irish Sea, September 2023.
Figure 8.7. Concentrations (Bq l-1) of tritium in surface water from the Bristol Channel, September 2023.
Figure 8.8. Concentrations (Bq l-1) of tritium in surface water from the English Channel, March - April 2023.
A seawater survey of the Irish Sea was carried out in 2023. As in previous surveys, a band of slightly higher concentrations of caesium‑137 was observed along the coast to the north and south of Sellafield, with levels generally decreasing with distance from the coast (Figure 8.4). The 2023 survey recorded concentrations of up to 0.04Bq l-1 in the eastern Irish Sea (in comparison with 0.04Bq l-1 in the eastern Irish Sea in 2021). For the remainder of the Irish Sea, caesium-137 concentrations were reported as less than values (0.02Bq l-1). Overall, concentrations were similar to those reported in the previous Irish Sea survey in 2021 (Environment Agency and others, 2020). Caesium-137 concentrations in the Irish Sea were only a very small percentage of those prevailing in the late 1970s, when discharges were substantially higher (typically up to 30Bq l-1 (Baxter, Camplin & Steele 1992)).
The predominant source of caesium-137 to the Irish Sea is considered to be remobilisation into the water column from activity associated with seabed sediment (Hunt, Leonard & Hughes 2013). Discharges from Sellafield have decreased substantially since the commissioning of the SIXEP waste treatment process in the mid-1980s, and this has been reflected in a decrease in caesium-137 concentrations in shoreline seawater at St Bees (Figure 8.9). In more recent years, the rate of decline of caesium‑137 concentrations over time has been decreasing at St Bees. Longer time series showing peak concentrations in the Irish Sea, and, with an associated time-lag, the North Sea are also shown in Figure 8.9.
Over several decades, the impact of discharges from the reprocessing plants at Sellafield and La Hague has been readily apparent, carried by the prevailing residual currents from the Irish Sea and the Channel, respectively (Povinec and others, 2003). Caesium-137 concentrations in the North Sea have tended to follow the temporal trends of the discharges, albeit with a time lag. The maximum discharge of caesium-137 occurred at Sellafield in 1975, with concentrations of caesium-137 of up to 0.5Bq l-1 in the North Sea surface waters in the late 1970s. Due to significantly decreasing discharges after 1978, remobilisation of caesium-137 from contaminated sediments in the Irish Sea was considered to be the dominant source of water contamination for most of the North Sea (McCubbin and others, 2002).
Figure 8.9. Concentration of caesium-137 in the Irish Sea, North Sea and in shoreline seawater close to Sellafield at St. Bees. (Note different scales used for activity concentrations).
In 2022, very low concentrations (up to 0.004Bq l-1) were found throughout most of the North Sea survey area (Environment Agency, Food Standards Agency and others, 2021). The few positively detected values were only slightly above those observed for global fallout levels in surface seawaters (0.0001 to 0.0028Bq l-1, (Povinec and others, 2005)). The overall distribution in the North Sea is characteristic of that observed in previous surveys over the last decade, with generally positively detected values near the coast, due to the long-distance transfer, possibly from Sellafield or Chernobyl-derived activity. In 2022, there was no significant evidence of input of Chernobyl-derived caesium-137 from the Baltic (via the Skagerrak) close to the Norwegian Coast. Trends and observations of caesium-137 concentrations in the waters of the North Sea (and Irish Sea), over the period 1995 to 2015, have been published (Leonard and others,. 2017).
In 2022, caesium-137 concentrations were reported as less than values (or close to the less than value) in the western English Channel (including those near the Channel Islands) and were not distinguishable from the background of fallout from nuclear weapons testing (Figure 8.5).
A full assessment of historic long-term trends of caesium-137 in surface waters of Northern European seas is provided elsewhere (Povinec and others, 2003).
Tritium concentrations in Irish Sea seawater in 2023 are shown in Figure 8.6, reported as less than values (or close to the less than value). As expected, these are generally higher (by small amounts) than those observed in the North Sea in 2022 (Figure 8.6, (Environment Agency and others, 2022)) due to the influence of discharges from Sellafield and other nuclear licensed sites. As in previous Irish Sea surveys, tritium concentrations to the south and west of the Isle of Man, including along the coastline of Ireland, were mostly reported as below (or close to) a less than value.
In the Bristol Channel, the combined effect of historical tritium discharges from the former GE Healthcare Limited facility at Cardiff[footnote 16], and those from Berkeley, Oldbury, and Hinkley Point, is shown in Figure 8.7. Tritium concentrations in the Bristol Channel were very low in 2023 (all below 5Bq l-1). Most results are reported as less than values (or close to the less than value) in the vicinity of the Welsh coast. Overall, tritium concentrations were lower in the inner region of the Bristol Channel, in comparison to recent years. There is no evidence of tritium entering the Irish Sea from the combined effect of discharges from the former GE Healthcare Limited facility at Cardiff, Berkeley, Oldbury, and Hinkley Point. Discharges from the Pharmaron UK radiolabelling facility, in Cardiff, also provide a minor contribution of tritium to this area. Tritium concentrations in the western English Channel were all reported as below the less than value (or close to the less than value) (Figure 8.8).
Technetium-99 concentrations in seawater have decreased following the substantial reduction in discharges resulting from Environment Agency requirements for discharge abatement. This followed substantial increases observed from 1994 to their most recent peak in 2003. The results of research cruises to study this radionuclide have been published (K. Leonard and others, 1997; K. S. Leonard and others, 1997; McCubbin and others, 2002; Leonard and others, 2004; Leonard and others, 2008) and an estimate of the total inventory residing in the sub-tidal sediments of the Irish Sea has also been published (Jenkinson and others, 2014). Trends in plutonium and americium concentrations in seawater of the Irish Sea have also been published (Leonard and others, 1999).
Full reviews of the quality status of the north Atlantic and a periodic evaluation of progress towards internationally agreed targets have been published by OSPAR (OSPAR 2000b; OSPAR 2009; OSPAR 2010; OSPAR 2016). The fifth periodic evaluation covers both radioactive discharges from the nuclear and non-nuclear sectors and environmental concentrations and demonstrated that Contracting Parties successfully fulfilled the RSS objectives for the nuclear and non-nuclear sectors (OSPAR 2022).
Shoreline sampling was also carried out around the UK, as part of routine site and regional monitoring programmes. Much of the shoreline sampling was directed at establishing whether the impacts of discharges from individual sites are detectable. Where appropriate, these are reported in the relevant sections of this report, and the results are collated in Table 8.10. Most radionuclides are reported as less than values. Tritium and caesium-137 concentrations remote from site discharge points are consistent with those in Figure 8.4 to Figure 8.8
Collection and analysis of marine sediment and seawater samples from across Scotland was not performed in 2023. Results up to 2019 are included in earlier RIFE reports (for example, (Environment Agency and others, 2020)).
9 References
Allott, R., 2005, Assessment of compliance with the public dose limit. Principles for the assessment of total retrospective public doses. NDAWG/2/2005., Chilton.
Baxter, A. & Camplin, W.C., 1994, ‘The use of caesium-137 to measure dispersion from discharge pipelines at nuclear sites in the UK’, Proceedings of the Institution of Civil Engineers - Water, Maritime and Energy, 106, 281–288.
Baxter, A., Camplin, W.C. & Steele, A.K., 1992, Radiocaesium in the seas of northern Europe: 1975 – 79, Lowestoft.
Brenk, H., Onishi, Y., Simmonds, J. & Subbaratnam, T., no date, A practical methodology for the assessment of individual and collective radiation doses in the environment. Draft working document number 1987-05-06, Vienna.
Brown, J. & Etherington, G., 2011, Health Risks from Radioactive Objects on Beaches in the Vicinity of the Sellafield Site. HPA-CRCE-018., Chilton.
Brown, J., Hammond, D., Wilding, D., Wilkins, B.T. & Gow, C., 2009, Transfer of radioactivity from seaweed to terrestrial foods and potential radiation exposures to members of the public: HPA-RPD-059, Chilton.
Byrom, J., Robinson, C.A., Simmonds, J.R., Walters, C.B. & Taylor, R.R., 1995, ‘Food consumption rates for use in generalised radiological dose assessments’, Journal of Radiological Protection, 15(4), 335–342.
Camplin, W.C., Grzechnik, M.P. & Smedley, C., 2005, Methods for assessment of total dose in the Radioactivity in Food and the Environment report. National Dose Assessment Working Group number 3, Chilton.
Camplin, W.C. & Jenkinson, S., 2007, Use of measurements in determining retrospective dose assessments in Radioactivity in Food and the Environment report. National Dose Assessment Working Group number 11/03, Chilton.
Clyne, F., 2021, Radiological Habits Survey: Metals Recycling Facility, 2018. RL 04/19, Lowestoft.
Clyne, F., Garrod, C. & Dewar, A., 2016, Radiological Habits Survey: Harwell, 2015. number RL 03/16, Lowestoft.
Clyne, F., Garrod, C., Dewar, A., Greenhill, B. & Ly, V., 2017, Radiological Habits Survey: Amersham, 2016. RL 02/17, Lowestoft.
Clyne, F., Garrod, C. & Ly, V., 2016, Radiological Habits Survey: Bradwell, 2015. number RL 02/16, Lowestoft.
Clyne, F., Garrod, C. & Papworth, G., 2015, Radiological Habits Survey: Berkeley and Oldbury, 2014. number RL 02/15, Lowestoft.
Clyne, F., Gough, C., Edgar, A., Garrod, C. & Elliott, J., 2010, Radiological Habits Survey: Sellafield Beach Occupancy, 2009. Project C3635 number RL 01/10, Lowestoft.
Clyne, F., Gough, C., Edgar, A. & Smedley, C., 2008, Radiological Habits Survey: Sellafield Beach Occupancy, 2007. Project C3015 number RL 02/08, Lowestoft.
Clyne, F.J., Greenhill, B.J., Moore, K.J., Mickleburgh, F.C. & Limbach, H.G., 2023, Radiological Habits Survey: Springfields, 2022. RL 05/23, Lowestoft.
Coleby, M., 2024, Personal Communication.
Commission of the European Community, 1987, ‘Council regulation (Euratom) number 3954/87 laying down the maximum permitted levels of radioactive contamination of foodstuffs and feeding stuffs following a nuclear accident or any other case of radiological emergency’, Official Journal of the European Union, (volume 11(L371), amended by Council Regulation 2218/89, 1989, Volume 1(L211)).
Commission of the European Community, 1992, ‘Council Directive 92/43/EEC of 21 May 1992 on the conservation of natural habitats and of wild fauna and flora’, Official Journal of the European Union, L206, 7–50.
Commission of the European Community, 1996, ‘Council Directive 96/29/Euratom of 13 May 1996 laying down basic safety standards for the protection of the health of workers and the general public against the dangers arising from ionising radiation’, Official Journal of the European Union, 39(L159), 1–114.
Commission of the European Community, 2000, ‘Commission recommendation on the application of Article 36 of the Euratom Treaty concerning the monitoring of the concentrations of radioactivity in the environment for the purpose of assessing the exposure of the population as a whole. 2000/473/Euratom’, Official Journal of the European Union.
Commission of the European Community, 2009, ‘Directive 2009/147/EC of the European Parliament and of the Council of 130 November 2009 on the conservation of wild birds’, Official Journal of the European Union, L 20, 7–25.
Committee of Radioactive Waste Management, 2023a, CoRWM Annual Report 2023 Nineteenth Annual Report 2023.
Committee of Radioactive Waste Management, 2023b, Position Paper: CoRWM consideration UK uranium inventory management disposal options.
Committee of Radioactive Waste Management, 2024, DEVELOPMENT OF SMALL MODULAR REACTORS AND ADVANCED MODULAR REACTORS-IMPLICATIONS FOR THE MANAGEMENT OF HIGHER ACTIVITY WASTES AND SPENT FUEL DEVELOPMENT OF SMALL MODULAR REACTORS AND ADVANCED MODULAR REACTORS-IMPLICATIONS FOR THE MANAGEMENT OF HIGHER ACTIVITY WASTES AND SPENT FUEL.
Committee on Interagency Research and Policy Coordination Alimentarius Commission, 2011, Codex Alimentarius Commission Report, Fact sheet on Codex guideline levels for radionuclides in food contaminated following a nuclear or radiological emergency.
Committee on Radioactive Waste Management, 2023, Committee on Radioactive Waste Management CoRWM Doc.3792 2 PROPOSED PROGRAMME OF WORK 2022.
Cooper, J.R., 2008, Review of risks from tritium – report of the AGIR – November 2007. Letter dated 17th April 2008, Chilton.
Corbett, J., 1983, ‘The Radiation Dose from Coal Burning: A Review of Pathways and Data’, Radiation Protection Dosimetry, 4(1), 5–19.
D. Harrison, J., Khursheed, A. & E. Lambert, B., 2002, ‘Uncertainties in Dose Coefficients for Intakes of Tritiated Water and Organically Bound Forms of Tritium by Members of the Public’, Radiation Protection Dosimetry, 98(3), 299–311.
Dale, I., Smith, P., Tyler, A., Copplestone D, Varley, A., Bradley, S. & Bartie, P., 2021, Radiological Habits Survey: Dounreay, 2018, Stirling.
Dale, I., Smith, P., Tyler, A., Copplestone D, Varley, A., Bradley, S., Bartie, P., Clarke, M. & Blake, M., 2021, Radiological Habits Survey: Hunterston 2017, Stirling.
Dale, P., Robertson, I. & Toner, M., 2008, ‘Radioactive particles in dose assessments’, Journal of Environmental Radioactivity, 99(10), 1589–1595.
Defence Science and Technology Laboratory, 2023, Marine Environmental Radioactivity at Nuclear Submarine Berths 2021.
Department for Business Energy & Industrial Strategy, Department for Environment Food & Rural Affairs, Welsh Government & Department of Agriculture Environment and Rural Affairs, 2018, Scope of and Exemptions from the Radioactive Substances Legislation in England, Wales, and Northern Ireland Guidance document, London, Cardiff and Belfast.
Department for Business Energy and Industrial Strategy, 2018, UK strategy for radioactive discharges; 2018 Review of the 2009 Strategy, London.
Department for Energy Security & Net Zero, Scottish Government, Department of Agriculture Environment and Rural Affairs & Welsh Government, 2024, UK policy framework for managing radioactive substances and nuclear decommissioning.
Department for Energy Security and Net Zero, 2023a, Consultation: Part I UK policy proposals for managing radioactive substances and nuclear decommissioning.
Department for Energy Security and Net Zero, 2023b, Consultation: Part II Draft UK policy framework for managing radioactive substances and nuclear decommissioning.
Department for Energy Security and Net Zero, 2024, Civil Nuclear : Roadmap to 2050, London.
Department for Environment Food & Rural Affairs, 2002, UK strategy for radioactive discharges 2001-2020.
Department for Environment Food & Rural Affairs, 2004, Contribution of aerial radioactive discharges to radionuclide concentrations in the marine environment. number DEFRA/RAS/04.002, London.
Department for Environment Food & Rural Affairs, 2010, Charting Progress 2, London.
Department for Environment Food & Rural Affairs, 2021, Marine Strategy Part Two: UK updated monitoring programmes.
Department for Environment Food & Rural Affairs, Department of Business Enterprise and Regulatory Reform, Welsh Assembly Government & Northern Ireland Assembly, 2008, Managing Radioactive Waste Safely A Framework for Implementing Geological Disposal, 2008. number Cm7386, London.
Department for Environment Food & Rural Affairs, Department of the Environment Northern Ireland, Scottish Government & Welsh Government, 2014, Marine Strategy Part Two: UK Marine Monitoring Programmes, London.
Department for Environment Food & Rural Affairs, Department of the Environment Northern Ireland, Scottish Government & Welsh Government, 2015, Marine Strategy Part Three: UK programme of measures, London.
Department for Environment Food & Rural Affairs, Department of the Environment Northern Ireland, Scottish Government & Welsh Government, 2019, Marine Strategy Part One: UK updated assessment and Good Environmental Status, London.
Department for Environment Food & Rural Affairs, Department of Trade and Industry & the Devolved Administrations, 2007, Policy for the Long-Term Management of Solid Low Level Radioactive Waste in the United Kingdom’, London.
Department of Agriculture Environment and Rural Affairs, Scottish Executive & Welsh Assembly Government, 2002, Safeguarding our seas. A strategy for the conservation and sustainable development of our marine environment, London.
Department of Business Energy and Industrial Strategy (BEIS), 2018a, Implementing Geological Disposal – Working With Communities, London.
Department of Business Energy and Industrial Strategy (BEIS), 2018b, Environmental Protection Act 1990: Part IIA Radioactive Contaminated Land Statutory Guidance, London.
Department of Business Energy and Industrial Strategy (BEIS), 2020, Energy White Paper: Powering our Net Zero Future, Department, vol. 44, London.
Department of Business Enterprise and Regulatory Reform, 2008, Meeting the energy challenge: A white paper in Nuclear Power. Cmnd 7296.
Department of Energy & Climate Change, 2011, Planning for new energy infrastructure Volume I of II.
Department of Energy and Climate Change, 2012, Environmental Protection Act 1990: Part IIA. Contaminated Land. Statutory Guidance, London.
Department of Energy and Climate Change, 2014, Implementing Geological Disposal, London.
Department of Energy and Climate Change, Department of the Environment Northern Ireland, Scottish Executive & Welsh Assembly Government, 2009, UK Strategy for Radioactive Discharges, London.
Department of Energy and Climate Change, Government, S., Welsh Government & Department of the Environment Northern Ireland, 2016, UK Strategy for the Management of Solid Low Level Radioactive Waste from the Nuclear Industry, London.
Department of the Environment Transport and the Regions, 2000, Radioactive Substances (Basic Safety Standards) (England and Wales) Direction 2000, London.
Dewar, A., Camplin, W., Barry, J. & Kennedy, P., 2014, ‘A statistical approach to investigating enhancement of polonium-210 in the Eastern Irish Sea arising from discharges from a former phosphate processing plant’, Journal of Environmental Radioactivity, 138, 289–301.
Dick, R., 2012, Personal Communication, Reading.
Dounreay Particles Advisory Group, 2008, 4th Report, November 2008, Stirling.
EDF Energy, 2018, Direct Radiation Dose to the Public from EDF Energy Nuclear Power Stations, 2015 to 2017. number ERO/REP/0197/GEN (as updated), Gloucester.
Environment Agency, 2002, Radioactivity In The Environment. Report for 2001, Lancaster.
Environment Agency, 2003, Radionuclides handbook. R&D Technical Report P3-101/SP1b, Bristol.
Environment Agency, 2006a, Initial radiological assessment methodology – part 1 user report. Number SC030162/SR1, Environment Agency, Bristol and London.
Environment Agency, 2006b, Initial radiological assessment methodology – part 2 methods and input data. Number SC030162/SR2, Bristol and London.
Environment Agency, 2008, Sellafield Radioactive Particles in the Environment – Programme of Work, February 2008, Bristol and London.
Environment Agency, 2009a, Habitats assessment for radioactive substances. Science report SC060083/SR1, May 2009, Bristol.
Environment Agency, 2009b, Impact of radioactive substances on Ribble and Alt estuarine habitats. Science report SC060083/SR2, Bristol.
Environment Agency, 2012a, Guidance Note for Developers and Operators of Radioactive Waste Disposal Facilities in England and Wales, Bristol and London.
Environment Agency, 2012b, Radioactive Contaminated Land, Bristol and London.
Environment Agency, 2020, Permit with introductory note. The Environmental Permitting (England and Wales) Regulations 2016. Sellafield Limited, Sellafield Site, Seascale, Cumbria, CA20 1PG. Variation notice number EPR/KP3690SX/VO11, permit number EPR/KP3690SX, Bristol.
Environment Agency, 2021, Permit with introductory note. The Environmental Permitting (England and Wales) Regulations. Sellafield Limited variation notice number EPR/KP3690SX/V012.
Environment Agency, 2023, Sellafield Permit KP3690SX VO13 Permit with introductory note.
Environment Agency, Environment and Heritage Service, Food Standards Agency & Scottish Environment Protection Agency, 2007, Radioactivity in Food and the Environment, 2006, Bristol, Belfast, London and Stirling.
Environment Agency, Food Standards Agency, Food Standards Scotland, Natural Resources Wales, Northern Ireland Environment Agency & Scottish Environment Protection Agency, 2015, Radioactivity in Food and the Environment, 2014, Bristol, London, Aberdeen, Cardiff, Belfast and Stirling.
Environment Agency, Food Standards Agency, Food Standards Scotland, Natural Resources Wales, Northern Ireland Environment Agency & Scottish Environment Protection Agency, 2016, Radioactivity in Food and the Environment, 2015, Bristol, London, Aberdeen, Cardiff, Belfast and Stirling.
Environment Agency, Food Standards Agency, Food Standards Scotland, Natural Resources Wales, Northern Ireland Environment Agency & Scottish Environment Protection Agency, 2017, Radioactivity in Food and the Environment, 2016, Bristol, London, Aberdeen, Cardiff, Belfast and Stirling.
Environment Agency, Food Standards Agency, Food Standards Scotland, Natural Resources Wales, Northern Ireland Environment Agency & Scottish Environment Protection Agency, 2018, Radioactivity in Food and the Environment, 2017, Bristol, London, Aberdeen, Belfast, Cardiff and Stirling.
Environment Agency, Food Standards Agency, Food Standards Scotland, Natural Resources Wales, Northern Ireland Environment Agency & Scottish Environment Protection Agency, 2019, Radioactivity in Food and the Environment, 2018, Bristol, London, Aberdeen, Cardiff, Belfast and Stirling.
Environment Agency, Food Standards Agency, Food Standards Scotland, Natural Resources Wales, Northern Ireland Environment Agency & Scottish Environment Protection Agency, 2020, Radioactivity in Food and the Environment, 2019, Bristol, London, Aberdeen, Cardiff, Belfast and Stirling.
Environment Agency, Food Standards Agency, Food Standards Scotland, Natural Resources Wales, Northern Ireland Environment Agency & Scottish Environment Protection Agency, 2021, Radioactivity in Food and the Environment, 2020, Bristol, London, Aberdeen, Belfast, Cardiff and Stirling.
Environment Agency, Food Standards Agency, Food Standards Scotland, Natural Resources Wales, Northern Ireland Environment Agency & Scottish Environment Protection Agency, 2022, Radioactivity in Food and the Environment, 2021, Bristol, London, Aberdeen, Cardiff, Belfast and Stirling.
Environment Agency, Food Standards Agency, Food Standards Scotland, Northern Ireland Environment Agency, Natural Resources Wales & Scottish Environment Protection Agency, 2018, Radioactivity in Food and the Environment. 2017. RIFE 23, Bristol, London, Aberdeen, Belfast, Cardiff and Stirling.
Environment Agency, Food Standards Agency, Food Standards Scotland, Northern Ireland Protection Agency, Natural Resources Wales & Scottish Environment Protection Agency, 2019, Radioactivity in Food and the Environment. 2018. RIFE 24, Bristol, London, Aberdeen, Belfast, Cardiff and Stirling.
Environment Agency, Food Standards Agency, Natural Resources Wales, Northern Ireland Environment Agency & Scottish Environment Protection Agency, 2014, Radioactivity in Food and the Environment, 2013, Bristol, London, Cardiff, Belfast and Stirling.
Environment Agency, Food Standards Agency, Northern Ireland Environment Agency, Natural Resources Wales & Scottish Environment Protection Agency, 2014, Radioactivity in Food and the Environment. 2013. RIFE 19., Bristol, London, Belfast, Cardiff and Stirling.
Environment Agency, Food Standards Agency, Northern Ireland Environment Agency & Scottish Environment Protection Agency, 2011, Radioactivity in Food and the Environment, 2010, Bristol, Belfast, London and Stirling.
Environment Agency, Food Standards Agency, Northern Ireland Environment Agency & Scottish Environment Protection Agency, 2012, Radioactivity in Food and the Environment, 2011, Bristol, London, Belfast and Stirling.
Environment Agency, Food Standards Agency, Northern Ireland Environment Agency & Scottish Environment Protection Agency, 2013, Radioactivity in Food and the Environment, 2012, Bristol, London, Belfast and Stirling.
Environment Agency, Food Standards Agency, Scotland, F.S., Natural Resources Wales, Agency, N.I.E. & Agency, S.E.P., 2016, Radioactivity in Food and the Environment, 2015, Bristol, London, Aberdeen, Cardif, Belfast and Stirling.
Environment Agency, Food Standards Agency & Scottish Environment Protection Agency, 2010, Environmental Radiological Monitoring. Radiological Monitoring Technical Guidance Note 2, Bristol, London and Stirling.
Environment Agency & Northern Ireland Environment Agency, 2009, Geological Disposal Facilities on Land for Solid Radioactive Wastes: Guidance on Requirements for Authorisation, Bristol and Belfast.
Environment Agency, Northern Ireland Environment Agency & Scottish Environment Protection Agency, 2009, Near-surface disposal facilities on land for solid radioactive wastes: guidance on requirements for authorisation, Bristol, Belfast and Stirling.
Environment Agency, Office for Nuclear Regulation, Natural Resources Wales & Scottish Environment Protection Agency, 2021, Regulatory Arrangements for the Management of Higher Activity Radioactive Waste on Nuclear Licensed Sites. Regulatory Position Statements - 2021 Update.
Environment Agency, Scottish Environment Protection Agency, Northern Ireland Environment Agency, Health Protection Agency & Food Standards Agency, 2012, Principles for the Assessment of Prospective Public Doses arising from Authorised Discharges of Radioactive Waste to the Environment Radioactive Substances Regulation under the Radioactive Substances Act (RSA-93) or under the Environmental Permitting Regulations (EPR-10), Bristol, Stirling, Belfast, Chilton and London.
Etherington, G., Youngman, M.J., Brown, J. & Oatway, W., 2012, Evaluation of the Groundhog Synergy Beach Monitoring System for Detection of Alpha-rich Objects and Implications for the Health Risks to Beach Users. HPA-CRCE-038., Chilton.
European Commission, 2013, ‘Council Directive 2013/51/EURATOM of 22 October 2013 laying down requirements for the protection of the health of the general public with regard to radioactive substances in water intended for human consumption’, Official Journal of the European Union L 296/12, (November 1998), 12–21.
European Commission, 2014, ‘Council Directive 2013/59/Euratom of 5 December 2013 laying down basic safety standards for protection against the dangers arising from exposure to ionising radiation, and repealing Directives 89/618/Euratom, 90/641/Euratom, 96/29/Euratom, 97/43/Euratom a’, Off J Eur Commun L13, (December 2003), 1–73.
European Commission, 2022, Commission Implementing Regulation (EU) 2021/1533 of 17 September 2021 imposing special conditions governing the import of feed and food originating in or dispatched from Japan following the accident at the Fukushima nuclear power station and repealing Implementing Regulation (EU) 2016/6 (Text with EEA relevance).
Food Standards Agency, 2001, Consultative Exercise on Dose Assessment, 3 and 4 October 2000. FSA/0022/0501.500, London.
Food Standards Agency, 2003, Analysis of farmed salmon for technetium-99 and other radionuclides. Food Survey Information Sheet Number 39/03, London.
Food Standards Agency, 2009, Estimate of the Food Chain Risks to Inform an Assessment of the Need for and Extent of the Food and Environment Protection Act Area at Dounreay, Aberdeen.
Food Standards Agency & Scottish Environment Protection Agency, 2002, Radioactivity in Food and the Environment, 2001, London and Stirling.
Garrod, C., Clyne, F., Greenhill, B. & Moran, C., 2017, Radiological Habits Survey: Heysham, 2016. RL 01/17, Lowestoft.
Garrod, C., Clyne, F. & Papworth, G., 2015, Radiological Habits Survey: Hartlepool, 2014/ RL 01/15, Lowestoft.
Garrod, C., Clyne, F. & Papworth, G., 2016, Radiological Habits Survey: Sizewell 2015, Lowestoft.
Garrod CJ, Clyne, F., Rumney, P. & Papworth, G., 2013a, Radiological Habits Survey: Barrow and the south-west Cumbrian coast, 2012. RL 01/13, Lowestoft.
Garrod CJ, Clyne, F., Rumney, P. & Papworth, G., 2013b, Radiological Habits Survey: Barrow and the south-west Cumbrian coast, 2012. RL 01/13, Lowestoft.
Graven, H.D. & Gruber, N., 2011, ‘Continental-scale enrichment of atmospheric 14CO2 from the nuclear power industry: potential impact on the estimation of fossil fuel-derived CO2’, Atmospheric Chemistry and Physics, 11(23), 12339–12349.
Greenhill, B., Clyne, F., Milligan, A. & Neish, A., 2018, Radiological Habits Survey: Hinkley Point, 2017. RL 09/18, Lowestoft.
Greenhill, B., Clyne, F. & Moore, K., 2019, Radiological Habits Survey: Trawsfynydd, 2018. RL 01/19, Lowestoft.
Greenhill, B., Clyne, F. & Moore, K., 2022, Radiological Habits Survey: Derby, 2021. RL 01/22, Lowestoft.
Greenhill, B., Clyne, F., Moore, K. & Mickleburgh, F., 2020, Radiological Habits Survey: Dungeness, 2019. RL 01/20, Lowestoft.
Greenhill, B.J., Clyne, F.J., Moore, K.J., Limbach, H.G. & Mickleburgh, F.C., 2024, Radiological Habits Survey: Wylfa, 2023 Cefas contract C8490.
Greenhill, B.J., Moore, K.J., Clyne, F.J., Limbach, H.G. & Mickleburgh, F.C., 2023, Radiological Habits Survey: Aldermaston and Burghfield, 2022. RL 04/23, Lowestoft.
Harrison, J.D. & Phipps, A., 2001, ‘Gut transfer and doses from environmental technetium’, Journal of Radiological Protection, 21(1), 9–11.
Harvey, M., Smith, J. & Cabianca, T., 2010, Assessment of collective and per caput doses due to discharges of radionuclides from the oil and gas industry into the marine environment. RPD-EA-4-2010, Chilton.
Health and Safety Executive, 2018, Work with ionising radiation. Ionising Radiations Regulations 2017: Approved Code of Practice and guidance. L121 (second edition), published 2018. ISBN 978 0 7176 6662 1, Norwich.
Health Protection Agency, 2007, Review of Risks from Tritium, London.
Health Protection Agency, 2009, Application of the 2007 Recommendations of the ICRP to the UK. Advice from the HPA, London.
Her Majesty’s Government, 2012, UK Initial Assessment and Good Environmental Status. December 2012, London.
Her Majesty’s Inspectorate of Pollution, 1995, Routine measurement of gamma ray air kerma rate in the environment. Technical Guidance Note (Monitoring) M5, London.
HM Government, 2022, British Energy Security Strategy, London.
Hodgson, A., Scott, J.E., Fell, T.P. & Harrison, J.D., 2005, Doses from the consumption of Cardiff Bay flounder containing organically bound tritium. Project SC020042/SR, vol. 25, Bristol.
Hughes, L., Runacres, S. & Leonard, K., 2011, Marine Radioactivity in the Channel Islands, 1990 – 2009’ Environmental Radiochemical Analysis 2011, Environmental Radiochemical Analysis 2011 volume IV, 170–180.
Hunt, G. & Rumney, H., 2004, The human gut transfer of environmental polonium-210. Proc. Int. Conf. on widening the radiation protection world, 23 – 28 May 2004, Madrid, Fontenay-aux-Roses.
Hunt, G. & Rumney, H., 2005, The human alimentary tract transfer of environmental polonium-210. Proceedings of the Seventh International Symposium of the Society for Radiological Protection, 12th-17th June 2005, Cardiff’, London.
Hunt, G.J., 1984, ‘Simple Models for Prediction of External Radiation Exposure from Aquatic Pathways’, Radiation Protection Dosimetry, 8(4), 215–224.
Hunt, G.J., 1998, ‘Transfer across the human gut of environmental plutonium, americium, cobalt, caesium and technetium: studies with cockles (Cerastoderma edule) from the Irish Sea’, Journal of Radiological Protection, 18(2), 101–109.
Hunt, G.J. & Allington, D.J., 1993, ‘Absorption of environmental polonium-210 by the human gut’, Journal of Radiological Protection, 13(2), 119–126.
Hunt, G.J., Hewett, C.J. & Shepherd, J.G., 1982, ‘The identification of critical groups and its application to fish and shellfish consumers in the coastal area of the north-east Irish Sea’, Health Physics, 43(6), 875–899.
Hunt, G.J., Leonard, D.R.P. & Lovett, M.B., 1986, ‘Transfer of environmental plutonium and americium across the human gut’, Science of The Total Environment, 53(1), 89–109.
Hunt, G.J., Leonard, D.R.P. & Lovett, M.B., 1990, ‘Transfer of environmental plutonium and americium across the human gut: A second study’, Science of The Total Environment, 90, 273–282.
Hunt, G.J. & Rumney, H.S., 2007, ‘The human alimentary tract transfer and body retention of environmental polonium-210’, Journal of Radiological Protection, 27(4), 405–426.
Hunt, G.J., Young, A.K. & Bonfield, R.A., 2001, ‘Transfer across the human gut of environmental technetium in lobsters ( Homarus gammarus L.) from the Irish Sea’, Journal of Radiological Protection, 21(1), 21–29.
Hunt, J., Bailey, T. & Reese, A., 2009, ‘The human body retention time of environmental organically bound tritium’, Journal of Radiological Protection, 29(1), 23–36.
Hunt, J., Leonard, K. & Hughes, L., 2013, ‘Artificial radionuclides in the Irish Sea from Sellafield: remobilisation revisited’, Journal of Radiological Protection, 33(2), 261–279.
International Atomic Energy Agency, 1996, International Basic Safety Standards for Protection against Ionizing Radiation and for the Safety of Radiation Sources, International Atomic Energy Agency, Vienna.
International Atomic Energy Agency, 1997, The joint convention on the safety of spent fuel management and on the safety of radioactive waste management: INFCIRC/546., Vienna.
International Atomic Energy Agency, 1999, Application of Radiological Exclusion and Exemption Principles to Sea Disposal The Concept of ‘de minimis’ for Radioactive Substances under the London Convention 1972, International Atomic Energy Agency, Vienna.
International Atomic Energy Agency, 2003, Determining the Suitability of Materials for Disposal at Sea Under the London Convention 1972: A Radiological Assessment Procedure, International Atomic Energy Agency, Vienna.
International Atomic Energy Agency, 2015, Determining the Suitability of Materials for Disposal at Sea under the London Convention 1972 and London Protocol 1996: A Radiological Assessment Procedure, International Atomic Energy Agency, Vienna.
International Commission on Radiological Protection, 1991, ‘1990 Recommendations of the International Commission on Radiological Protection’, Annals of the ICRP, 21(1–3).
International Commission on Radiological Protection, 1994, ‘Age-dependent Doses to Members of the Public from Intake of Radionuclides - Part 2 Ingestion Dose Coefficients’, Annals of the ICRP, 23(3–4), 1.
International Commission on Radiological Protection, 2001, ‘Doses to the Embryo and Fetus from Intakes of Radionuclides by the Mother’, Annals of the ICRP, 31(1–3).
International Commission on Radiological Protection, 2007, The 2007 Recommendations of the International Commission on Radiological Protection, vol. 37.
International Commission on Radiological Protection, 2008, ‘Environmental Protection - the Concept and Use of Reference Animals and Plants’, Annals of the ICRP, 38(4–6).
International Commission on Radiological Protection, 2010, ‘Conversion Coefficients for Radiological Protection Quantities for External Radiation Exposures’, Annals of the ICRP, 40(2–5).
International Commission on Radiological Protection, 2012, ‘ICRP publication 119: Compendium of dose coefficients based on ICRP publication 60’, Annals of the ICRP, 41.
International Commission on Radiological Protection, 2014, Protection of the Environment under Different Exposure Situations, vol. 43.
International Organisation for Standardisation, 2017, General requirements for the competence of testing and calibration laboratories. number 17025.
Jenkinson, S.B., McCubbin, D., Kennedy, P.H.W., Dewar, A., Bonfield, R. & Leonard, K.S., 2014, ‘An estimate of the inventory of technetium-99 in the sub-tidal sediments of the Irish Sea’, Journal of Environmental Radioactivity, 133, 40–47.
Jobling, S., Williams, R., Johnson, A., Taylor, A., Gross-Sorokin, M., Nolan, M., Tyler, C.R., Aerle, R. van, Santos, E. & Brighty, G., 2006, ‘Predicted Exposures to Steroid Estrogens in U.K. Rivers Correlate with Widespread Sexual Disruption in Wild Fish Populations’, Environmental Health Perspectives, 114(Suppl 1), 32–39.
Jones, A. & Harvey, M.P., 2014, Radiological Consequences Resulting from Accidents and Incidents Involving the Transport of Radioactive Materials in the UK - 2012 Review. PHE-CRCE-014, Chilton.
Jones, K., Smith, J., Anderson, T., Harvey, M., Brown, I., Field, S. & Jones, A., 2013, Implied doses to the population of the EU arising from reported discharges from EU nuclear power stations and reprocessing sites in the years 2004 to 2008. RP 176, Publications Office.
Kershaw, P. & Baxter, A., 1995, ‘The transfer of reprocessing wastes from north-west Europe to the Arctic’, Deep Sea Research Part II: Topical Studies in Oceanography, 42(6), 1413–1448.
Knowles, J.F., Smith, D.L. & Winpenny, K., 1998, ‘A Comparative Study of the Uptake, Clearance and Metabolism of Technetium in Lobster (Homarus Gammarus) and Edible Crab (Cancer Paguras)’, Radiation Protection Dosimetry, 75(1–4), 125–129.
Kocher, D.C. & Eckerman, K.F., 1987, ‘Electron Dose-rate Conversion Factors for External Exposure of the Skin From Uniformly Deposited Activity on the Body Surface’, Health Physics, 53(2).
Leonard, K., Donaszi-Ivanov, A., Dewar, A., Ly, V. & Bailey, T., 2017, ‘Monitoring of caesium-137 in surface seawater and seafood in both the Irish and North Seas: trends and observations’, Journal of Radioanalytical and Nuclear Chemistry, 311(2), 1117–1125.
Leonard, K., McCubbin, D., Brown, J., Bonfield, R. & Brooks, T., 1997, ‘A summary report of the distribution of Technetium-99 in UK Coastal Waters’, Radioprotection, 32, 109–114.
Leonard, K., McCubbin, D., Jenkinson, S., Bonfield, R. & McMeekan, I., 2008, An assessment of the availability of Tc-99 to marine foodstuffs from contaminated sediments. Project R01062. RL09/08, Lowestoft.
Leonard, K.S., McCubbin, D., Blowers, P. & Taylor, B.R., 1999, ‘Dissolved plutonium and americium in surface waters of the Irish Sea, 1973-1996’, JOURNAL OF ENVIRONMENTAL RADIOACTIVITY, 44(2–3), 129–158.
Leonard, K.S., McCubbin, D., Brown, J., Bonfield, R. & Brooks, T., 1997, ‘Distribution of technetium-99 in UK coastal waters’, MARINE POLLUTION BULLETIN, 34(8), 628–636.
Leonard, K.S., McCubbin, D., McDonald, P., Service, M., Bonfield, R. & Conney, S., 2004, ‘Accumulation of technetium-99 in the Irish Sea?’, Science of The Total Environment, 322(1–3), 255–270.
Limited, B.N.F., 2002, Discharges and monitoring of the environment in the UK. Annual Report 2001, Warrington.
Low Level Waste Repository Limited, 2018, LLWR Plan 2018-2023, Holmrook.
Ly, V., Cogan, S., Camplin, W., Peake, L. & Leonard, K., 2015, Long Term Trends in far-field effects of marine radioactivity measured around Northern Ireland. ERA12: Proceedings of the International Symposium on Nuclear and Environmental Radiochemical Analysis (17-19 September 2014, Bath, UK), Cambridge.
McCubbin, D., Leonard, K.S., Brown, J., Kershaw, P.J., Bonfield, R.A. & Peak, T., 2002, ‘Further studies of the distribution of technetium-99 and caesium-137 in UK and European coastal waters’, Continental Shelf Research, 22(10), 1417–1445.
McCubbin, D. & Vivian, C., 2006, Dose assessments in relation to disposal at sea under the London Convention 1972: judging de minimis radioactivity. For Defra Project AA005. RL 05/06, Lowestoft.
McKay, W., Barr, H., Halliwell, C., Spencer, D., Adsley, I. & Perks, C., 1995, Site specific background dose rates in coastal areas. DoE/HMIP/RR/94/037, London.
Ministry of Agriculture FIsheries and Food, 1993, Terrestrial Radioactivity Monitoring Programme (TRAMP) report for 1992.
Ministry of Agriculture Fisheries and Food, 1995, Terrestrial Radioactivity Monitoring Programme (TRAMP) Report for 1994, London.
Ministry of Agriculture Fisheries and Food, 1996, Pesticides Safety Directorate’s Handbook. Appendix IC, London.
Ministry of Agriculture Fisheries and Food & Scottish Environment Protection Agency, 1998, Radioactivity in Food and the Environment, 1997, London and Stirling.
Ministry of Agriculture Fisheries and Food & Scottish Environment Protection Agency, 1999, Radioactivity in Food and the Environment, 1998, London and Stirling.
Mobbs, S., Barraclough, I. & Napier, I., 1998, A review of the use and disposal of gaseous tritium light devices, United Kingdom.
Moore, K., Clyne, F. & Greenhill, B., 2020, Radiological Habits Survey: Winfrith 2019. RL09/20, Lowestoft.
Moore, K., Clyne, F. & Greenhill, B., 2022, Radiological Habits Survey: Capenhurst, 2021. RL 02/22, Lowestoft.
Moore, K., Clyne, F., Greenhill, B. & Clarke, K., 2018, Radiological Habits Survey: Devonport, 2017. RL 10/18, Lowestoft.
Moore, K.J., Clyne, F.J. & Greenhill, B.J., 2023, Radiological Habits Survey: Sellafield Review, 2022. RL 01/23, Lowestoft.
Moore, K.J., Greenhill, B.J., Mickleburgh, F.C., Limbach, H.G. & Clyne, F.J., 2024a, Radiological Habits Survey: Sellafield, 2023 Cefas contract C8490.
Moore, K.J., Greenhill, B.J., Mickleburgh, F.C., Limbach, H.G. & Clyne, F.J., 2024b, Radiological Habits Survey: Low Level Waste Repository, 2023 Cefas contract C8490.
Moore, K.J., Greenhill, B.J., Mickleburgh, F.C., Limbach, H.G. & Clyne, F.J., 2024c, Radiological Habits Survey: Sellafield, 2023 Cefas contract C8490.
National Dose Assessment Working Group, 2004, Radiological Assessment Exposure Pathways Checklist (Common and Unusual). NDAWG/2/2004, Chilton.
National Radiological Protection Board, 1990, ‘Gut transfer factors’, Docs NRPB, 1(2).
National Radiological Protection Board, 2005, Guidance on the application of dose coefficients for the embryo and fetus from intakes of radionuclides by the mother. Docs NRPB 16(2), Chilton.
Natural Scotland & Scottish Environment Protection Agency, 2016, Radioactive Substances Unit Part IIA Inspection and Risk Assessment Report Site: Alienated Land Former RAF Kinloss, Edinburgh.
Northern Ireland Assembly, 2003, The Radioactive Substances (Basic Safety Standards) Regulations (Northern Ireland) 2003, 33(208).
Nuclear Decommissioning Agency, 2023, NDA Mission Progress Report, Moor Row, Cumbria.
Nuclear Decommissioning Agency, 2024, Nuclear Decommissioning Authority Business Plan 2024 - 2027.
Nuclear Decommissioning Authority, 2010, UK Strategy for the Management of Solid Low Level Radioactive Waste from the Nuclear Industry, Moor Row, Cumbria.
Nuclear Decommissioning Authority, 2020, NDA Business Plan 2020 to 2023. SG/2020/58, Moor Row, Cumbria.
Nuclear Decommissioning Authority, 2021, Strategy: Effective from March 2021, Moor Row, Cumbria.
Nuclear Decommissioning Authority & Department for Business Energy and Industrial Strategy, 2019, 2019 UK Radioactive Waste Detailed Data, Moor Row, Cumbria.
Oatway, W. & Brown, J., 2015, Health Risk to Seafood Consumers from Radioactive Particles in the Marine Environment near Sellafield Public Health England. PHE-CRCE-021, Chilton.
Oatway, W., Cabianca, T. & Jones, A., 2020, Assessing the risk to people’s health from radioactive objects on beaches around the Sellafield site Summary report. PHE-CRCE-056, Chilton.
Oatway, W.B., Jones, A.L., Holmes, S., Watson, S. & Cabianca, T., 2016, Ionising radiation exposure of the UK population: 2010 Review. PHE-CRCE-026, Chilton.
Office for Nuclear Regulation, Natural Resources Wales, Scottish Environment Protection Agency & Environment Agency, 2021, The management of higher activity radioactive waste on nuclear licensed sites.
Organisation for Economic Co-operation and Development Nuclear Energy Agency, 1985, Review of the continued suitability of the dumping site for radioactive waste in the North-East Atlantic, Paris.
OSPAR, 1998, SINTRA Statement. Summary Record OSPAR 98/14/1, Annex 45, London.
OSPAR, 2000a, Convention for the protection of the marine environment of the North-East Atlantic, London.
OSPAR, 2000b, Quality Status Report 2000, London.
OSPAR, 2007, Second Periodic Evaluation of progress towards the objective of the Radioactive Substances Strategy.
OSPAR, 2009, Towards the Radioactive Substances Strategy Objectives Third Periodic Evaluation, London.
OSPAR, 2010, Quality Status Report 2010, London.
OSPAR, 2016, Towards the Radioactive Substances Strategy Objectives. Fourth Periodic Evaluation, London.
OSPAR, 2017, OSPAR Coordinated Environmental Monitoring Programme (CEMP) (OSPAR Agreement 2016-01). CEMP Appendix R1 and R2, London.
OSPAR, 2021a, Strategy of the OSPAR Commission for the Protection of the North-East Atlantic 2030, London.
OSPAR, 2021b, Cascais Declaration. Ministerial meeting of the OSPAR Commission October 2021, London.
OSPAR, 2022, Towards the Radioactive Substances Strategy Objectives. Fifth Periodic Evaluation., London.
OSPAR, 2024a, Summary Record. Meeting of the Radioactive Substances Committee (RSC). Lisbon 6th - 9th February 2024., London.
OSPAR, 2024b, Radioactive Substances Series Annual report and assessment of discharges of liquid discharges from nuclear installations in 2022, London.
OSPAR, 2024c, Radioactive Substances Series Annual report and assessment of discharges of radionuclides from the non-nuclear sectors in 2022, London.
Papworth, G., Garrod, C. & Clyne, F., 2014, Radiological Habits Survey: Dounreay, 2013. RL 06/14, Lowestoft.
Particles Retrieval Advisory Group (Dounreay), 2010, Annual Report to SEPA and DSRL, March 2010, Stirling.
Particles Retrieval Advisory Group (Dounreay), 2011, Annual Report to SEPA and DSRL, March 2011, Stirling.
Particles Retrieval Advisory Group (Dounreay), 2016, Annual report to SEPA and DSRL, SEPA, Stirling. March 2016, Stirling.
Povinec, P.P., Aarkrog, A., Buesseler, K.O., Delfanti, R., Hirose, K., Hong, G.H., Ito, T., Livingston, H.D., Nies, H., Noshkin, V.E., Shima, S. & Togawa, O., 2005, ‘90Sr, 137Cs and 239,240Pu concentration surface water time series in the Pacific and Indian Oceans – WOMARS results’, Journal of Environmental Radioactivity, 81(1), 63–87.
Povinec, P.P., Bailly du Bois, P., Kershaw, P.J., Nies, H. & Scotto, P., 2003, ‘Temporal and spatial trends in the distribution of 137Cs in surface waters of Northern European Seas—a record of 40 years of investigations’, Deep Sea Research Part II: Topical Studies in Oceanography, 50(17), 2785–2801.
Preston, A., Mitchell, N.T. & Jefferies, D.F., 1974, Experience gained in applying the ICRP critical group concept to the assessment of public radiation exposure in control of liquid radioactive waste disposal, IAEA, International Atomic Energy Agency (IAEA).
Rollo, S., Camplin, W., Duckett, L., Lovett, M. & Young, A., 1994, Airborne radioactivity in the Ribble Estuary. pp277 – 280. In: Proc. IRPA Regional Congress on Radiological Protection, 6 – 10 June 1994, Portsmouth, UK.
Rollo, S.F.N., Camplin, W.C., Allington, D.J. & Young, A.K., 1992, ‘Natural Radionuclides in the UK Marine Environment’, Radiation Protection Dosimetry, 45(1–4), 203–209.
Scotland & Northern Ireland Forum for Environmental Research, 2005, A Review of the Application of ‘Best Practicable Means’ within a Regulatory Framework for Managing Radioactive Wastes, Edinburgh.
Scottish Environment Protection Agency, 2007, Strategy for the Assessment of the potential impact of Sellafield Radioactive Particles on Southwest Scotland, Stirling.
Scottish Environment Protection Agency, 2012, SEPA Policy on the Regulation of Disposal of Radioactive Low-Level Waste from Nuclear Sites’, Stirling.
Scottish Environment Protection Agency, 2017, Guidance on Monitoring for Heterogeneous Radium-226 Sources Resulting from Historic Luminising or Waste Disposal Sites, Stirling.
Scottish Environment Protection Agency, 2019a, Environmental Radiological Monitoring in Scotland Radiological Monitoring Technical Guidance Note 2 Reviewed October 2019, Stirling.
Scottish Environment Protection Agency, 2019b, Satisfying the optimisation requirement and the role of Best Practicable Means. RS-POL-001 Version 2.0, Stirling.
Scottish Environment Protection Agency, 2020, Guidance on decommissioning of non- nuclear facilities for radioactive substances activities. Version 3.0, Stirling.
Scottish Environment Protection Agency, no date, Radiological Habits Survey: Torness 2023. In Press.
Scottish Environment Protection Agency, no date, Radiological Habits Survey: Chapelcross, 2022. In press.
Scottish Environment Protection Agency, no date, Radiological Habits Survey: Faslane 2023. In Press.
Scottish Environment Protection Agency, no date, Radiological Habits Survey: Rosyth 2022. In Press.
Scottish Environment Protection Agency, Environment Agency & Natural Resources Wales, 2018, Management of radioactive waste from decommissioning of nuclear sites: Guidance on Requirements for Release from Radioactive Substances Regulation V.1.0. July 2018, Stirling, Bristol and Cardiff.
Scottish Executive, 2000, The Radioactive Substances (Basic Safety Standards) (Scotland) Regulations 2000 (revoked), Edinburgh.
Scottish Executive, 2006, Environmental Protection Act 1990: Part IIA Contaminated Land. Statutory Guidance: Edition 2. Scottish Executive number SE/2006/44, Edinburgh.
Scottish Government, 2009, Environmental Protection Act 1990: Part IIA Contaminated Land. The Radioactive Contaminated Land (Scotland) Regulations 2007 Statutory Guidance’ Scottish Government number SG/2009/87, Edinburgh.
Scottish Government, 2018, The Environmental Authorisations (Scotland) Regulations 2018. Scottish Statutory Instruments, Edinburgh.
Scottish Statutory Instruments, 2015, The Private and Public Water Supplies (Miscellaneous Amendments) (Scotland) Regulations 2015.
Simmonds, J., Lawson, G. & Mayall, A., 1995, Radiation Protection 72: Methodology for assessing the radiological consequences of routine releases of radionuclides to the environment. EUR 15760, Luxenbourg.
Smith, B. & Jeffs, T., 1999, Transfer of radioactivity from fishmeal in animal feeding stuffs to man. RL 8/99, Lowestoft.
Smith, D., Smith, B., Joyce, A. & McMeekan, I., 2002, An assessment of aquatic radiation exposure pathways in Northern Ireland. SR(02)14. RL 20/02, Lowestoft.
Smith, J.G.. & Simmonds, J.R.. (Editors), 2009, The methodology for assessing the radiological consequences of routine releases of radionuclides to the environment used in PC-CREAM 08: HPA-RPD-058, Health Protection Agency, Chilton.
Smith, K.R. & Jones, A.L., 2003, ‘Generalised Habit Data for Radiological Assessments, NRPB-W41’.
Smith, P., Dale, I., Tyler, A., Copplestone D, Varley, A., Bradley, S., Bartie, P., Clarke, M. & Blake, M., 2021, Radiological Habits Survey: Dumfries & Galloway Coast 2017, Stirling.
Statutory Instruments, 2007, SI 2007 No. 3236. The Radioactive Contaminated Land (Northern Ireland) (Amendment) Regulations 2007.
Statutory Instruments, 2010, 2010 No. 2145. The Radioactive Contaminated Land Regulations (Northern Ireland) (Amendment) Regulations 2010.
Statutory Instruments, 2016, SI 2016 No. 614. The Water Supply (Water Quality) Regulations 2016.
Statutory Rules of Northern Ireland, 2017, The Water Supply (Water Quality) Regulations (Northern Ireland) 2017, 52.
Statutory Rules of Northern Ireland, 2018, SR 2018 No 116. The Radioactive Substances (Modification of Enactments) Regulations (Northern Ireland) 2018.
Swift, D.J. & Nicholson, M.D., 2001, ‘Variability in the edible fraction content of 60Co, 99Tc, 110mAg, 137Cs and 241Am between individual crabs and lobsters from Sellafield (north eastern Irish Sea)’, Journal of Environmental Radioactivity, 54(3), 311–326.
Thurston, L. & Gough, C., 1992, Investigation of radiation exposure pathways from liquid effluents at Holy Loch: Local Habits Survey 1989. RL 7/92’, Lowestoft.
UK Statutory Instruments, 2018a, SI 2018 No. 1278. The Ionising Radiation (Basic Safety Standards) (Miscellaneous Provisions) (Amendment) (EU Exit) Regulations 2018.
UK Statutory Instruments, 2018b, SI 2018 No 428 The Ionising Radiation (Basic Safety Standards) (Miscellaneous Provisions) Regulations 2018.
UK Statutory Instruments, 2019, The Conservation of Habitats and Species (Amendment) (EU Exit) Regulations 2019.
United Kingdom - Parliament, 1965, Nuclear Installations Act, 1965.
United Kingdom - Parliament, 1985, Food and Environment Protection Act 1985.
United Kingdom - Parliament, 1993, Radioactive Substances Act, 1993.
United Kingdom - Parliament, 1995a, Environment Act 1995.
United Kingdom - Parliament, 1995b, Review of Radioactive Waste Management Policy: Final Conclusions, London.
United Kingdom - Parliament, 1999a, The Ionising Radiations Regulations 2017. Statutory Instrument 2017 number 1075.
United Kingdom - Parliament, 1999b, The Ionising Radiations Regulations 1999. Stat. Inst. 1999 No 3232.
United Kingdom - Parliament, 2004, Energy Act 2004.
United Kingdom - Parliament, 2009, Marine and Coastal Access Act 2009.
United Kingdom - Parliament, 2016, Environmental Permitting (England and Wales) Regulations. Stat. Inst. 2016 No 1154.
United Kingdom - Parliament, 2018, Environmental Permitting (England and Wales) (Amendment) (No. 2) Regulations. Stat. Inst. 2018 No 428.
United Kingdom - Parliament, 2020a, The Environmental Permitting (England and Wales) (Amendment) (EU Exit) Regulations 2019, 1(39).
United Kingdom - Parliament, 2020b, The Waste and Environmental Permitting etc. (Legislative Functions and Amendment etc.) (EU Exit) Regulations 2020. Statutory Instrument 1540.
Watson, S., Jones, A., Oatway, W. & Hughes, J., 2005, Radiation Exposure of the UK Population: 2005 Review. HPA-RPD-001, Chilton.
Welsh Statutory Instruments, 2018, The Water Supply (Water Quality) Regulations 2018, 60.
Young, A., McCubbin, D., Thomas, K., Camplin, W., Leonard, K. & Wood, N., 2003, 210Po Concentrations in UK Seafood, in P. Warwick (ed.), 9th International Symposium on Environmental Radiochemical Analysis, 18-20 September 2002. Oxford, ERA II, 143–149, Royal Society of Chemistry, London.
Young, A.K., McCubbin, D. & Camplin, W.C., 2002, Natural Radionuclides in Seafood. Project R03010/C0808. RL 17/02, Lowestoft.
Appendix 1 Disposals of radioactive waste
Tables A1.1 to A1.5 downloadable in Open Document Spreadsheet (ODS) Format on the main RIFE page
Summary of unintended leakages, spillages, emissions or unusual findings of radioactive substances from nuclear licensed sites in the UK in 2023 (see Table A1.5 in the ODS file)
Sellafield, November 2019
Summary of incident
Sellafield Limited notified ONR and the Environment Agency that the liquor balance model for the Magnox Swarf Storage Silo (MSSS) indicated an ongoing loss of contaminated water from the MSSS building. This is an update to an on-going event that commenced in 2019 (see Table A2.5, RIFE 27 2022).
Consequences and actions taken
There is an ongoing leak of radioactive liquid from the MSSS facility at Sellafield into the ground. Sellafield Ltd estimate that the leak started in July 2019. They formally reported it to the Environment Agency in November 2019.
The cause of the leak is believed to be reopening of historical cracks in the facility structure that caused leakage to ground in the 1970s. When the leak resumed in 2019, its rate initially increased up to around 2,400 litres per day. It has stabilised at this level since October 2020.
The Environment Agency expect the leak to result in significant long-term consequence due to additional radioactive contamination of the ground and groundwater at Sellafield. This will need to be addressed in the clean-up of the site, with potentially significant implications for:
- nuclear liabilities
- the quantities of low and intermediate level waste resulting from the site clean-up
Based on the current understanding of the leak, the Environment Agency conclude that any risk to the off-site environment and public:
- is expected to be very low
- would be realised over an extended timescale
There is:
- no risk to public water supply boreholes from the leak
- no risk of drawing any contaminated groundwater towards these boreholes
The Environment Agency have classified the leak as category 1 (major) on our compliance and common incident classification schemes because of the long-term consequence.
The Environment Agency’s formal investigation into the resumption of the leak resulted in a warning letter to Sellafield Limited in July 2022. The continued leakage represents an ongoing non-compliance with the permit. The Environment Agency recognise that there are significant challenges in addressing the leak because of the nature of the facility and its location. Therefore, the Environment Agency’s regulatory focus is on working with Sellafield Ltd to ensure an effective and timely plan is implemented to address the leak.
As part of our regulatory response, in March 2021 the Environment Agency issued a series of improvement requirements for the MSSS (as part of a further variation to the permit).
The improvement requirements in the permit require action to tackle the MSSS leak. They aim to:
- stop or minimise the current leak and its consequences
- minimise the potential for further below ground leaks in the future
In July 2023 Sellafield Limited provided the Environment Agency with its detailed plan to meet these improvement requirements. The plan includes decisions to be taken on:
- how and when to retrieve waste from the silos
- possible implementation of measures in the silo and in ground to reduce the consequences of the leak
The Environment Agency assessed the plan and agreed to its delivery on 4 December 2023. Sellafield Limited is now required by conditions we introduced into its permit in response to the leak to implement the plan.
The Environment Agency is working closely with ONR in response to the leak. You can read ONR’s report about the MSSS leak.
Read Sellafield Ltd’s case study about the MSSS.
Sellafield, May 2023
Summary of incident
Environment Agency were notified of a discharge to ground of potentially contaminated rainwater from the Highly Active Liquor Evaporation and Storage (HALES) Buffer Storage Tank Facility Roof, HALES Buffer Storage Export Plant Flask and HALES Transfer Tower Drip Tray.
Consequences and actions taken
The Environment Agency determined that there were breaches of the permit in allowing a potential disposal of radioactive waste from an unpermitted disposal route and for not maintaining the HALES Buffer Storage Tank Facility Roof Drainage and the ventilation ductwork in good repair.
The Environment Agency determined these both Category 3 non-compliances and the environmental impact of the event was considered to be minor. The Environment Agency agreed that the actions already put in place by Sellafield Limited are sufficient to minimise recurrence and set no further actions.
Hartlepool, Q1 2020, Q1, 2021, Q1 2022, Q1 2023
Summary of incident
The Environment Agency were notified in June 2024 that EDF Energy Nuclear Generation Ltd have been under-reporting the activity concentrations in the 30mm core samples due to the wrong units being used (Bq kg-1 rather than Bq m-2). No notification thresholds or permit limits have been breached.
Consequences and actions taken
EDF E NGL have discovered a recurring mistake in the Q1 quarterly returns for core samples between 2020 – 2023 (inclusive). The core results are only reported once per year in the Q1 returns
The 30mm core samples are reported in Bq m-2 but EDF E NGL have been reporting results in Bq kg-1 (the same units that the 5mm samples are reported in). The use of the wrong units started between the 2019 and 2020 reporting period.
The results have been recalculated using the correct documented method and the below table summarises the differences between the reported and actual results (plus error margins for the methods used). These data are presented in the Appendix 1 tables.
EDF E NGL have confirmed that no results from this time period have exceeded the mean plus 3 or 4 standard deviations notification threshold. The Environment Agency is reviewing the data and investigating the root causes of this minor potential breach of EDF E NGL’s environmental permit.
Appendix 2 Abbreviations and Glossary
ABL
AWE plc, Babcock and Lockheed Martin UK
AGIR
Advisory Group on Ionising Radiation
AGR
Advanced Gas-cooled Reactor
AMR
Advanced Modular Reactor
AWE
Atomic Weapons Establishment
BAT
Best Available Techniques
BEIS
Department of Business, Energy and Industrial Strategy
BNFL
British Nuclear Fuels plc
BPM
Best Practicable Means
BSS
Basic Safety Standards
BSSD 13
Basic Safety Standards 2013
CCFE
Culham Centre for Fusion Energy
CEDA
Consultative Exercise on Dose Assessments
Cefas
Centre for Environment, Fisheries & Aquaculture Science
COMARE
Committee on Medical Aspects of Radiation in the Environment
CoRWM
Committee on Radioactive Waste Management
DAERA
Department of Agriculture Environment and Rural Affairs
DCO
Development Consent Order
Defra
Department for Environment, Food and Rural Affairs
DESNZ
Department for Energy Security & Net Zero
DPAG
Dounreay Particles Advisory Group
DSRL
Dounreay Site Restoration Limited
DWPF
Decommissioning Waste Processing Facility
Euratom
European Atomic Energy Community
EASR18
Environmental Authorisations (Scotland) Regulations 2018
EARP
Enhanced Actinide Removal Plant
EC
European Commission
EIA
Environmental Impact Assessment
ENRMF
East Northants Resource Management Facility
EPR
Environmental Permitting Regulations
EPR 16
Environmental Permitting (England and Wales) Regulations 2016
EPR 18
Environmental Permitting (England and Wales) Regulations 2018
EPR 19
Environmental Permitting (England and Wales) Regulations 2019
EPRTM
European Pressurised Reactor™
EU
European Union
FEPA
Food and Environment Protection Act
FSA
Food Standards Agency
FSS
Food Standards Scotland
GDA
Generic Design Assessment
GDF
Geological Disposal Facility
GES
Good Environmental Status
GOCO
Government Owned Contractor Operator
GRR
Guidance on Requirements for Release of Nuclear Sites from Radioactive Substances Regulation
HAW
Higher Activity Radioactive Waste
HEPA
High-Efficiency Particulate Filters
HMIP
His Majesty’s Inspectorate of Pollution
HMNB
His Majesty’s Naval Base
HPA
Health Protection Agency
HSE
Health and Safety Executive
IAEA
International Atomic Energy Agency
ICRP
International Commission on Radiological Protection
ID
Indicative Dose
ILW
Intermediate Level Waste
ILWS
Intermediate Level Waste Store
IRPA
International Radiation Protection Association
IRR 17
Ionising Radiations Regulations 2017
ISO
International Standards Organisation
JET
Joint European Torus
JWMP
Joint Waste Management Plan
LLLETP
Low Level Liquid Effluent Treatment Plant
LLW
Low-Level Waste
LLWF
Low Level Radioactive Waste Facility
LLWR
Low Level Waste Repository
LoA
Letter of Agreement
LoD
Limit of Detection
MAFF
Ministry of Agriculture, Fisheries & Food
MAST
Mega Amp Spherical Tokamak
MCAA
Marine Coastal Access Act 2009
MMO
Marine Management Organisation
MOD
Ministry of Defence
MRF
Metals Recycling Facility
MRL
Minimum Reporting Level
MSSS
Magnox Swarf Storage Silo
NCA
Nuclear Cooperation Agreement
ND
Not Detected
NDA
Nuclear Decommissioning Authority
NDAWG
National Dose Assessment Working Group
NEAES
North-East Atlantic Environment Strategy
NIEA
Northern Ireland Environment Agency
NNL
National Nuclear Laboratory
NORM
Naturally Occurring Radioactive Material
NRPB
National Radiological Protection Board
NRS
Nuclear Restoration Services Limited
NRW
Natural Resources Wales
NRTE
Naval Reactor Test Establishment
NWS
Nuclear Waste Services
OBT
Organically Bound Tritium
ONR
Office for Nuclear Regulation
OWPF
Operational Waste Processing Facility
OSPAR
Oslo and Paris Convention for the Protection of the marine environment of the North-East Atlantic
PBO
Parent Body Organisation
PCM
Plutonium Contaminated Materials
PRAG(D)
Particles Retrieval Advisory Group (Dounreay)
PHE
Public Health England
PWR
Pressurised Water Reactor
RAPs
Reference Animals and Plants
RDP
Repository Development Programme
RIFE
Radioactivity in Food and the Environment
RRDL
Rosyth Royal Dockyard Limited
RNAS
Royal Naval Air Station
RRSL
Rolls-Royce Submarines Limited
RSA 93
Radioactive Substances Act 1993
RSR
Radioactive Substances Regulation
RSR 18
Radioactive Substances (Modification of Enactments) Regulations (Northern Ireland) 2018
RSRL
Research Sites Restoration Limited
RSS
Radioactive Substances Strategy
RVS
Retrievals Ventilation System
RWM
Radioactive Waste Management Ltd
SAC
Special Areas of Conservation
SEPA
Scottish Environment Protection Agency
SFL
Springfields Fuels Limited
SILWE
Solid Intermediate Level Waste Encapsulation Plant
SIXEP
Site Ion Exchange Effluent Plant
SMR
Small Modular Reactor
SPA
Special Protection Areas
STW
Sewage Treatment Works
THORP
Thermal Oxide Reprocessing Plant
TENORM
Technologically Enhanced Naturally Occurring Radioactive Material
TRAMP
Terrestrial Radioactivity Monitoring Programme
UCP
Urenco ChemPlants Limited
UKAEA
United Kingdom Atomic Energy Authority
UKAS
UK Accreditation Service
UKHSA
UK Health Security Agency (formerly PHE and HPA)
UKNWM
UK Nuclear Waste Management Limited
UOC
Uranium Ore Concentrate
UNS
Urenco Nuclear Stewardship Limited
UUK
Urenco UK Limited
VLLW
Very Low-Level Waste
WILWREP
Wet Intermediate Level Waste Retrieval and Encapsulation
Absorbed dose
The ionising radiation energy absorbed in a material per unit mass. The unit for absorbed dose is the gray (Gy) which is equivalent to J kg-1.
Artificial radionuclides
These are radioactive isotopes that are not found readily in nature. They are generally produced during nuclear power generation, nuclear fuel reprocessing, from nuclear weapons testing or in specialist devices by neutron bombardment.
Authorised premises
These are premises that has been authorised by the environment agencies to discharge to the environment.
Becquerel
One radioactive transformation per second.
Bioaccumulation
Excretion may occur; however, the rate of excretion is less than the rate of intake + accumulation.
Biota
Flora and fauna.
Canteen meals
Canteen meals are used to represent a typical or mixed diet at locations remote from nuclear sites throughout the UK. Prior to leaving the EU, these data were collected and reported to comply with Articles 35 and 36 of Euratom. These samples are maintained to demonstrate compliance with Euratom recommendations. Additional information is provided in Section 2.1.3.
Committed effective dose
The sum of the committed equivalent doses for all organs and tissues in the body resulting from an intake (of a radionuclide), having been weighted by their tissue weighting factors. The unit of committed effective dose is the sievert (Sv). The ‘committed’ refers to the fact that the dose is received over a number of years, but it is accounted for in the year of the intake of the activity.
Direct radiation
Ionising radiation which arises directly from processes or operations on premises using radioactive substances and not as a result of discharges of those substances to the environment.
Dose
Shortened form of ‘effective dose’ or ‘absorbed dose’.
Dose limits
Maximum permissible dose resulting from ionising radiation from practices covered by the Euratom Basic Safety Standards Directive, excluding medical exposures. It applies to the sum of the relevant doses from external exposures in the specified period and the 50 year committed doses (up to age 70 for children) from intakes in the same period. Currently, the limit has been defined as 1mSv per year for the UK.
Dose rates
The radiation dose delivered per unit of time.
Effective dose
The sum of the equivalent doses from internal and external radiation in all tissue and organs of the body, having been weighted by their tissue weighting factors. The unit of effective dose is the sievert (Sv).
Environmental materials
Environmental materials include freshwater, grass, seawater, seaweed, sediment, soil and various species of plants.
Equivalent dose
The absorbed dose in a tissue or organ weighted for the type and quality of the radiation by a radiation-weighting factor. The unit of equivalent dose is the sievert (Sv).
External dose
Doses to humans from sources that do not involve ingestion or inhalation of the radionuclides.
Fragments
‘Fragments’ are considered to be fragments of irradiated fuel, which are up to a few millimetres in diameter.
Ionising radiation
Radiation composed of particles that individually carry enough kinetic energy to liberate an electron from an atom or molecule, ionising it. Ionising radiation is generated through nuclear reactions, either artificial or natural, by very high temperature (for example, plasma discharge or the corona of the Sun), via production of high energy particles in particle accelerators, or due to acceleration of charged particles by the electromagnetic fields produced by natural processes, from lightning to supernova explosions.
Indicator materials
Environmental materials may be sampled for the purpose of indicating trends in environmental performance or likely impacts on the food chain. These include seaweed, soil and grass.
In-growth
Additional activity produced as a result of radioactive decay of parent radionuclides.
Kerma air rate
Air kerma is the quotient of the sum of the kinetic energies of all the charged particles liberated by indirectly ionising particles in a specified mass of air.
Millisievert
The millisievert is a 1/1000 of a sievert. A sievert is one of the International System of Units used for the measurement of dose equivalent.
Nuclear Sites
Shortened form of ‘Nuclear Licensed sites’.
Orphan source
Is a radioactive source which is neither exempted nor under regulatory control. For example, this could be because it has never been under regulatory control or abandoned, lost misplaced, stolen or otherwise transferred without proper approval.
Radiation exposure
Being exposed to radiation from which a dose can be received.
Radiation weighting
Factor used to weight the tissue or organ absorbed dose to take account of the type and quality of the radiation. Example radiation weighting factors: alpha particles = 20; beta particles = 1; photons = 1.
Radioactivity
The emission of alpha particles, beta particles, neutrons and gamma or x-radiation from the transformation of an atomic nucleus.
Radionuclide
An unstable form of an element that undergoes radioactive decay.
Representative person
Representative person is an approach used in the assessment of radiation exposures (‘total doses’) to the public. Direct measurement of doses to the public is not possible under most normal conditions. Instead, doses to the public are estimated using environmental radionuclide concentrations, dose rates and habits data. The estimated doses are compared with dose criteria. In this report, the dose criteria are legal limits for the public.
Source specific dose
An assessment of dose that focuses on specific sources on sites (for example, liquid or gaseous discharges) and their associated pathways (for example, external exposure over shoreline areas). See Section 2, and Appendix 4 for more information on these dose calculations.
In some cases, assessments may include the impacts from multiple sites. For example, the source specific assessment of the Dumfries and Galloway coast includes the impacts of discharges from Chapelcross and Sellafield.
TENORM
Naturally occurring radioactive materials that may have been technologically enhanced in some way. The enhancement has occurred when a naturally occurring radioactive material has its composition, concentration, availability, or proximity to people altered by human activity. The term is usually applied when the naturally occurring radionuclide is present in sufficient quantities or concentrations to require control for purposes of radiological protection of the public or the environment.
Tissue weighting factors
Factor used to weight the equivalent dose in a tissue or organ to take account of the different radiosensitivity of each tissue and organ. Example tissue weighting factors: lung = 0.12; bone marrow = 0.12; skin = 0.01.
‘Total dose’
An assessment of dose that takes into account all exposure pathways such as radionuclides in food and the environment and direct radiation.
Appendix 3 Modelling of concentrations of radionuclides in foodstuffs, air, and sewage systems
Tables for this appendix are downloadable in Open Document Spreadsheet (ODS) Format on the main RIFE page.
A3.1 Foodstuffs
At Sellafield and the LLWR near Drigg, a simple food chain model has been used to provide concentrations of activity in milk and livestock for selected radionuclides to supplement data obtained by direct measurements. This is done where relatively high limits of detection exist or where no measurements were made.
Activities in milk, meat and offal were calculated for technetium-99, ruthenium-106, cerium-144, and plutonium-241 using the equations:
Cm = Fm Ca Qf and
Cf = Ff CaQf where
Cm is the concentration in milk (Bq l-1),
Cf is the concentration in meat or offal (Bq kg-1(fresh)),
Fm is the fraction of the animal’s daily intake by ingestion transferred to milk (d l-1)
Ff is the fraction of the animal’s daily intake by ingestion transferred to meat or offal (d kg-1 (fresh)),
Ca is the concentration in fodder (Bq kg-1 (dry)),
Qf is the amount of fodder eaten per day (kg (dry) d-1)
No direct account is taken of radionuclide decay or the intake by the animal of soil associated activity. The concentration in fodder is assumed to be the same as the maximum observed concentration in grass or, in the absence of such data, in leafy green vegetables. The food chain data for the calculations are given in Table A3.1 (Simmonds, Lawson & Mayall 1995; Brenk and others, unpublished) and the estimated concentrations in milk, meat and offal are presented in Table A3.2.
A3.2 Air
For some sites, discharges to air may lead to significant doses. Doses may arise from radionuclides transferred from the plume to food crops and animal products, inhalation of radionuclides in the plume itself and external doses from radionuclides in the plume.
Average annual concentrations of radionuclides in the air at nearest habitations were calculated using a Gaussian plume model, PC CREAM 08 (Smith and Simmonds, 2009), and the reported discharges of radionuclides to air. Each site assessment uses generic meteorological data based on the Pasquill stability category shown in Table A3.3. The core modelling assumptions (such as the effective discharge/stack height and distance to habitation) are also shown in Table A3.3. The discharge stack is assumed to be at the centre of the site. For multi-station sites (for example, Sizewell A and B), the gaseous discharges are summed together and assumed to be discharged via a common discharge point (at the centre of both sites).
External radiation doses from radionuclides in the plume and from deposited activity were calculated taking into account occupancy levels indoors and outdoors and location factors to allow for building shielding. During the time people are assumed to be indoors, the standard assumption that the dose from gamma-emitting radionuclides in the plume will be reduced by 80% has been made. Internal radiation doses from inhalation of discharged radionuclides were assessed using breathing rates. Doses were initially assessed for three age groups: adults, children (10-year-old), and infants (1-year-old). All ages are assumed to have year-round occupancy at the nearest habitation. The assumptions of the inhalation and occupancy rates assessment are shown in Table A3.4. The dose to the prenatal children age group was taken to be the same as that for an adult.
A3.3 Sewage systems
The facilities at Aldermaston and Amersham discharge liquid radioactive waste to local sewers. Wastes are processed at local sewage treatment works (STW). The prolonged proximity to raw sewage and sludge experienced by sewage treatment workers could lead to an increase in the dose received, via a combination of external irradiation from the raw sewage and sludge and the inadvertent ingestion and inhalation of resuspended radionuclides.
An assessment of the dose received by workers at the Maple Lodge STW, near Amersham and the Silchester STW, near Aldermaston has been conducted using the methodology and data given by the Environment Agency (Environment Agency 2006a; Environment Agency 2006b). The flow rate through the sewage works is used to calculate a mean concentration in raw sewage and sludge of each nuclide discharged. These mean concentrations are combined with habits data concerning the workers’ occupancy near raw sewage and sludge, external and internal dosimetric data, and physical data such as inhalation rates to provide dose estimates. Workers are assumed to spend 75% of a working year in proximity to the raw sewage, and the other 25% in proximity to the sewage sludge. Where liquid discharges are not nuclide-specific, a composition has been assumed based on advice from the operators and concentrations calculated accordingly.
The model parameters and habits data used to assess the dose to sewage treatment workers are given in Table A3.5 and the amounts of radioactivity discharged from each site can be found in Appendix 1 of this report.
Appendix 4 Consumption, inhalation, handling and occupancy rates
Tables for this appendix are downloadable in Open Document Spreadsheet (ODS) Format on the main RIFE page.
This appendix gives the consumption, handling and occupancy rate data used in the source specific assessment of exposures from terrestrial consumption and aquatic pathways. Consumption rates for terrestrial foods are based on (Byrom and others, 1995) and are given in Table A4.1. These are derived from national statistics and are taken to apply at each site. Site-specific data for aquatic pathways based on local surveys are given in Table A4.2. These site-specific data have been supplemented with referenceable generic information (Environment Agency 2002; Smith & Jones 2003) where appropriate. Occupancy over intertidal areas and rates of handling from local surveys has been reassessed to take account of a change in the factor used to determine the range of rates typical of those most exposed. For using the ‘cut-off’ method to define those most exposed (Preston, Mitchell & Jefferies 1974; Hunt, Hewett & Shepherd 1982) a factor of 3.0 is used to describe the ratio of the maximum to the minimum rate within the group. Data used for routine assessments of external and inhalation pathways from gaseous discharges are given in Appendix 3.
Consumption rates refer to the mass of a foodstuff as prepared for consumption (with, for example, stalks or shells removed) and are consistent with the mass quantity used for presentation of concentration data in this report. The term “fresh weight” is used in the data tables of concentrations. For shellfish, the consumption rates and concentrations are for cooked weights. For other foodstuffs, uncooked weights are used.
Appendix 5 Dosimetric data
Tables for this appendix are downloadable in Open Document Spreadsheet (ODS) Format on the main RIFE page.
The dose coefficients used in assessments in this report are provided in Table A5.1 for ease of reference. For adults and postnatal children, they are based on generic data contained in ICRP Publication 119 (International Commission on Radiological Protection 2012). Dose coefficients for prenatal children have been obtained primarily from ICRP 88 (International Commission on Radiological Protection 2001) and NRPB (National Radiological Protection Board 2005). For a few radionuclides where prenatal dose coefficients are unavailable the relevant adult dose coefficient has been used.
In the case of tritium, polonium, plutonium and americium radionuclides, dose coefficients have been adjusted according to specific research work of relevance to assessments in this report.
A5.1 Polonium
The current ICRP advice is that a gut uptake factor of 0.5 is appropriate for dietary intakes of polonium by adults (International Commission on Radiological Protection 1994). A study involving the consumption of crab meat containing natural concentrations of polonium-210 has suggested that the factor could be as high as 0.8 (Hunt & Allington 1993). More recently, similar experiments with mussels, cockles and crabs suggested a factor in the range 0.15 to 0.65, close to the ICRP value of 0.5 (Hunt & Rumney 2004; Hunt & Rumney 2005; Hunt & Rumney 2007). Previous assessments have considered the effects of a factor of 0.8 for considering monitoring results in RIFE. In view of the most recent review (Hunt & Rumney 2007), a value of 0.5 has been adopted for all food, consistent with ICRP advice.
A5.2 Plutonium and americium
Studies using adult human volunteers have suggested a gut uptake factor of 0.0002 is appropriate for the consumption of plutonium and americium in winkles from near Sellafield (Hunt, Leonard & Lovett 1986; Hunt, Leonard & Lovett 1990). For these and other actinides in food in general, a factor of 0.0005 is considered as a reasonable best estimate (National Radiological Protection Board 1990). These values are to be used if data are not available for the specific circumstances under consideration. In this report, a gut uptake factor of 0.0002 is used for plutonium and americium, for estimating doses to consumers of winkles from Cumbria and this is consistent with UKHSA advice. For other foods and for winkles outside Cumbria, the factor of 0.0005 is used for these radionuclides. This choice is supported by studies of cockle consumption (Hunt 1998).
A5.3 Technetium-99
Volunteer studies have been extended to consider the transfer of technetium-99 in lobsters across the human gut (Hunt, Young & Bonfield 2001). Although values of the gut uptake factor found in this study were lower than the ICRP value of 0.5, dose coefficients are relatively insensitive to changes in the gut uptake factor. This is because the effective dose is dominated by ‘first pass’ dose to the gut (Harrison & Phipps 2001). In this report, we have therefore retained use of the standard ICRP factor and dose coefficient for technetium-99.
A5.4 Tritium
In 2002, UKHSA reviewed the use of dose coefficients for tritium associated with organic material (D. Harrison, Khursheed & E. Lambert 2002). Subsequently, UKHSA published a study of the uptake and retention of organically bound tritium (OBT) in rats fed with fish from Cardiff Bay ((Hodgson and others, 2005). These experiments suggested that the dose coefficient for OBT in fish from the Severn Estuary near Cardiff should be 6.0E-11 Sv Bq-1, higher than the standard ICRP value for OBT ingestion. The higher value is used for adults in the assessment of seafood collected near the Cardiff site in this report, and the standard ICRP value for other assessments. This approach is consistent with advice (Cooper 2008) which takes account of the conclusions reached by the Independent Advisory Group on Ionising Radiation (AGIR) concerning relative biological effectiveness and radiation weighting (Health Protection Agency 2007). Thereafter, results of uptake experiments involving adult volunteers, who ate samples of sole from Cardiff Bay, provided further evidence that this approach is indeed cautious (Hunt, Bailey & Reese 2009).
Appendix 6 Estimates of concentrations of natural radionuclides
Tables for this appendix are downloadable in Open Document Spreadsheet (ODS) Format on the main RIFE page.
A6.1 Aquatic foodstuffs
Table A6.1 gives estimated values of concentrations of radionuclides due to natural sources in aquatic foodstuffs. The values are based on sampling and analysis conducted by Cefas (Young, McCubbin & Camplin 2002; Young and others, 2003). Data for lead-210 and polonium-210 are quoted as medians with minimum and maximum values given in brackets. Dose assessments for aquatic foodstuffs are based on concentrations of these radionuclides net of natural background.
The carbon-14 concentrations are adjusted to take account of the dilution of natural atmospheric carbon-14 by the emission of carbon dioxide from fossil fuel burning. A dilution of 0.28% for each part per million of carbon dioxide added due to fossil fuel burning is used (Graven & Gruber 2011). Values for the carbon dioxide additions are taken each year from: National Oceanic and Atmospheric Administration carbon dioxide annual mean data (in ppm).
The initial specific activity of carbon-14 was 256Bq kg-1 (Ministry of Agriculture Fisheries and Food 1995). In 2023, the adjusted value used as the basis for Table A6.1 was 211Bq kg-1.
A6.2 Terrestrial foodstuffs
The values of carbon-14 in terrestrial foodstuffs due to natural sources that are used in dose assessments are given in Table A6.2 and based on earlier data (Ministry of Agriculture Fisheries and Food 1995). The value for the specific activity of carbon-14 in 2022 (given in Section A6.1) was used to derive these estimates.
Appendix 7 Research in support of the monitoring programmes
The environment agencies, FSA and FSS have programmes of special investigations and supporting research and development studies to complement the routine monitoring programmes. This additional work is primarily directed at the following objectives:
- to evaluate the significance of potential sources of radionuclide contamination of the food chain and the environment
- to identify and investigate specific topics or pathways not currently addressed by the routine monitoring programmes and the need for their inclusion in future routine monitoring
- to develop and maintain site-specific habits and agricultural practice data, to ensure that dose assessment calculations reflect the real-world situation
- to develop more sensitive and/or efficient analytical techniques for measurement of radionuclides in natural matrices
- to evaluate the competence of laboratories’ radiochemical analytical techniques for specific radionuclides in food and environmental materials
- to develop improved methods for handling and processing monitoring data
Other studies include projects relating to effects on wildlife, emergency response and planning and development of new environmental models and data.
Information on ongoing and recently completed extramural research is presented in the list below (and Table A7.1, available from the main RIFE page. Those sponsored by the Environment Agency and FSA are also listed on their websites: Environment Agency, and Food Standards Agency, respectively. Copies of the final reports for each of the projects funded by the FSA are available from Clive House, 70 Petty France, London, SW1H 9EX. Further information on studies funded by SEPA is available by emailing [email protected]. Environment Agency reports are available from Environment Agency. A charge may be made to cover costs.
Extramural projects
- Soil and herbage survey, reference UKRSR01 and SCO00027, on behalf of the Environment Agency and SEPA, due Q4, 2025
- Offshore Dose Assessment Model, SEPA, due Q3, 2024
- Thorium Transfer Work, SEPA - the intent is to publish on the SEPA website, in accordance with accessibility requirements, in 2025
- NORM Biota Project, SEPA - the intent is to publish on the SEPA website, in accordance with accessibility requirements, in 2025
- McGuire, C (2023). Radiological Protection of the Public from Radioactive Particles (PhD Thesis). University of Stirling. SEPA. The official completion date of McGuire, C. (2023) is 25th January 2023. It will however be embargoed until 25th January 2025, when available in the University of Stirling repository. McGuire Thesis on Universoty of Stirling thesis repository
- Clyde Estuary Assessment, SEPA, due Q2, 2025
- Soil and herbage follow-up, SEPA, complete Q1, 2024
- Coastal Monitoring, SEPA - the intent is to publish on the SEPA website, in accordance with accessibility requirements, in 2025
- Shetland Background Sampling, SEPA - the intent is to publish on the SEPA website, in accordance with accessibility requirements, in 2025
- Partitioning Behaviour of Radionuclides in Sewer Systems, Environment Agency, due Q3, 2024
- Radionulide Transfer Parameter Review, Environment Agency, due Q4, 2024
- Investigation of the fate and behaviour of lutetium-177 in the environment, Environment Agency, due Q4 2024
-
International Commission on Radiological Protection (ICRP) recommendations (International Commission on Radiological Protection 2007) use the term ‘representative person’ for assessing doses to members of the public. It is defined as ‘an individual receiving a dose that is representative of the more highly exposed individuals in the population’. RIFE reports published before 2013 referred to an average dose to individuals in a group of people (the ‘critical group’) rather than to a single person. The ‘representative person’ concept is considered equivalent to the ‘critical group’. ↩
-
The Cumbrian coastal community are exposed to radioactivity resulting from both current and historical discharges from the Sellafield site and naturally occurring radioactivity discharged from the former phosphate processing works near Whitehaven, close to Sellafield. ↩
-
See Section 1.2, Appendix 2 and Appendix 5 for more information. ↩ ↩2
-
The Oslo and Paris Convention for the protection of the marine environment of the North-East Atlantic. ↩
-
‘Particles and objects’ are terms used which encompass discrete radioactive items which can range in radioactivity concentration, size and origin. ‘Particles’ include radioactive scale, fragments of irradiated nuclear fuel and incinerated waste materials (less than 2mm in diameter). ‘Objects’ are larger radioactive artefacts and stones which have radioactive contamination on their surface and are larger than 2mm in size. Particles can be compared according to the hazard posed. ↩
-
A hypothetical individual is used in radiological dose assessments as it is often not possible to identify a specific member of a population whose habits are likely to result in them receiving a dose towards the upper end of the range seen in that population when variability and uncertainty in exposure pathways and source of radiation are considered. Use of the concept of the representative person has been recommended by the ICRP and UKHSA as a practical approach to assess the radiological impact of exposure to a source of radiation. ↩
-
The Environment Agency has an agreement with Natural Resources Wales to undertake some specific activities on its behalf including radiological environmental monitoring and Radioactive Substances Regulation of nuclear sites in Wales. ↩
-
At some locations separate nuclear licensed sites are situated adjacent to one another, for example some EDF Energy operated power stations have a neighbouring decommissioning Magnox station. As these are operated by different employers, workers at one station are considered to be members of the public for the purpose of assessing direct radiation exposure to the other station. Doses to workers are considered differently to those for the public and therefore are not included in ‘total dose’ assessments. ↩
-
Natura 2000 is made up of sites designated as Special Areas of Conservation (SACs) and Special Protection Areas (SPAs). SACs and SPAs in the UK no longer form part of the EU’s Natura 2000 ecological network. The 2019 Regulations have created a national site network on land and at sea, including both the inshore and offshore marine areas in the UK. ↩
-
In England and Wales, the term ‘permit’ replaced ‘authorisation’ under the Environmental Permitting Regulations (EPR). In this report ‘permit’ has been used to apply to all sites in England and Wales, irrespective of whether the period considered includes activities prior to EPR coming into force in 2010. In Scotland, the term ‘permit’ replaced ‘authorisation’ under the Environmental Authorisations (Scotland) Regulations 2018 (EASR18), irrespective of whether the period includes activities prior to EASR18 coming into force in 2018. ‘Authorisation’ remains the relevant term for Northern Ireland. ↩
-
The treaty establishing the European Atomic Energy Community (Euratom) was signed in Rome on 25 March 1957. ↩
-
The reference to proprietary products in this report should not be construed as an official endorsement of those products, nor is any criticism implied of similar products which have not been mentioned. ↩ ↩2
-
The Cumbrian coastal community are exposed to radioactivity resulting from both current and historical discharges from the Sellafield site and naturally occurring radioactivity discharged from the former phosphate processing works near Whitehaven, close to Sellafield. ↩
-
Values are rounded to 2 significant figures, or 3 decimal places, depending on their magnitude. ↩
-
‘Particles and objects’ are terms used which encompass discrete radioactive items which can range in radioactivity concentration, size and origin. ‘Particles’ include radioactive scale, fragments of irradiated nuclear fuel and incinerated waste materials (less than 2mm in diameter). ‘Objects’ are larger radioactive artefacts and stones which have radioactive contamination on their surface and are larger than 2 mm in size. Particles can be compared according to the hazard posed. ↩
-
GE Healthcare ceased manufacturing tritium and carbon-14 radiolabelled compounds in 2009 and 2010, respectively. Its custom radiolabelling division was acquired by Quotient Bioresearch (Radiochemicals) Ltd in 2010, and subsequently by Pharmaron UK Ltd in 2017. This operation is located at a new premises in Cardiff under a non-nuclear permit, albeit at lower levels. ↩ ↩2 ↩3
-
The FEPA Order was made in 1997 following the discovery of fragments of irradiated nuclear fuel on the seabed near Dounreay, by United Kingdom atomic energy authority (UKAEA), and prohibits the collection of seafoods within a 2km radius of the discharge pipeline. ↩
-
DPAG was set up in 2000, and PRAG(D) thereafter, to provide independent advice to SEPA and UKAEA on issues relating to the Dounreay fragments. ↩